Every year, the unusual mix of minerals in the Hajar Mountains near the coast of Oman traps 100,000 tons of carbon in the rock.
That estimate is a tiny fraction of the potential of the mountain range and a few others like it in the world.
Based on decades of work by geologists studying this unique formation—known as the Samail Ophiolite—the highly reactive rocks called peridotites can theoretically trap one-half ton of CO2 per ton of that rock in the Hajar Mountains, which extend into the UAE.
A leading voice among the researchers who made the 100,000-ton estimate and developed ideas for removing trillions of tons of carbon from the atmosphere is Peter Kelemen, a professor at Columbia University in New York City.
In a 2019 story in Scientific American, Kelemen said that if it is possible to speed the pace of mineralization “by a factor of a million”—something he thinks is doable with a bit of engineering—“then you end up with a billion tons of CO2 per cubic kilometer of rock per year.”
That potential inspired an Omani entrepreneur, Talal Hasan, to start a company based on the work of Kelemen and colleagues including Juerg Matter, a geochemist now working at the University of Southampton in England. The result was startup 44.01, named for the molecular mass of carbon, where Kelemen serves as an advisor and Matter works part time.
The company’s website describes its plan: “Carbon mineralization in peridotite is happening all the time—we simply speed up the natural process.”
It is a simple-sounding goal. But what it will take to realize the vast potential is anything but simple. In an interview, Kelemen identified why: “The main concern is that the rocks are not very porous.”
Or very permeable, which helps explain why large amounts of highly reactive elements have remained untouched over the 96 million years these reactive minerals have been on land.
The hard rocks in this formation, particularly magnesium-rich olivine, were produced when magma flowed up from the mantle to a mid-ocean ridge where it cooled and the thick layer of rock spread out toward what is now Oman and the UAE, and something extraordinary happened.
When it reached the boundary between two plates, it normally would have returned to the depths of the earth. Instead, it collided with another ophioplite and was ultimately thrust up onto land.
When the theory of plate tectonics transformed geological thinking during the second half of the 20th century, this giant anomaly became a destination for geologists eager to study a rare outcrop of rock from the mantle, including Kelemen.
They also could have gone to New Guinea to look at a similar display of mantle rock or scattered sites in the western US, among other places, where ophiolites are present. But the Hajar Mountains comprise the biggest ophiolite of them all.
Speeding carbon mineralization from geological rates to the pace needed to help stop global warming poses a fundamental problem common to unconventional oil and gas—creating flow paths in tight rock on a limited budget.
Another similarity is that the descriptions of the potential of peridotites are reminiscent of the early days of the US shale revolution when the US Geological Survey issued reports showing the unconventional formations held mind-boggling volumes of oil and gas.
The size of these resource estimates turned out to be in line with reality, but the challenges of getting oil and gas in tiny pores to flow out of ultralow-permeability formations made getting more than 10% of the oil out an achievement.
Yet, what was produced was still enough to move global oil markets for years. Peridotite potential is also so large that tapping even a fraction of it could play a significant role in limiting greenhouse gas levels in the atmosphere.
In the Scientific American story, Kelemen estimated the worldwide CO2 storage capacity of these rocks at roughly 25 to 250 times the amount that humans have added to the atmosphere since 1850. It is reasonable to assume that actual development of storage will never reach all the reactive rock, but it is too early to say what the technical and economic limits will be.
Currently 44.01 is working on its third injection test in Fujairah, UAE, with backing from ADNOC—the national oil company owned by the government of Abu Dhabi.
The announcement for the project said “CO2 will be captured from the air, dissolved in seawater, and then injected into peridotite formations deep underground, where it will mineralize”—which is verb strongly preferred by 44.01, which objects to the word “stored,” because they consider storage in places like old oil and gas fields as not as secure.
The release added few details about the well or the injection program, but plenty has been written offering clues.
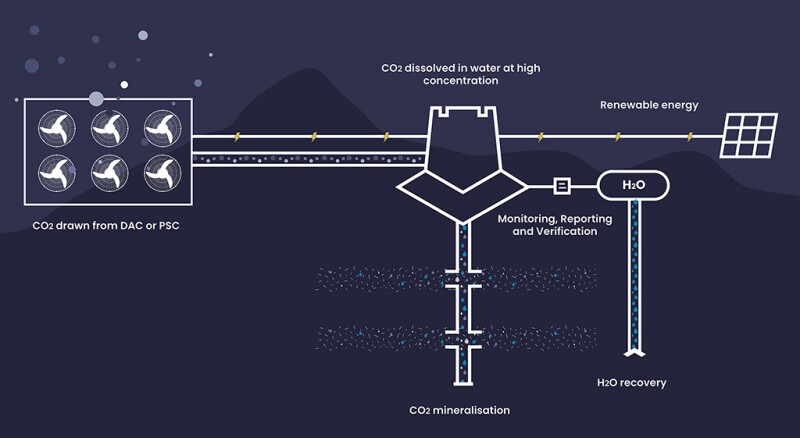
Chemical Reaction Cracking
Describing why it might be possible to lock trillions of tons of carbon in peridotite, Kelemen said in a story posted on Columbia University’s website, “We are inspired by the fact that in some places the rocks have naturally become fully carbonated. That is, every magnesium and calcium atom in them has combined with CO2 to create solid minerals.”
Those were likely sections of the rock that was flooded eons ago, putting the rock in contact with water and CO2 to drive reactions.
That is not normally the case. In test wells drilled so far, even the rock within a few hundred feet of the surface remains largely unaffected by the air and water above.
For example, core pulled from the upper 50–100 m of one test well showed many veins of carbonate, likely formed by reactions when rainwater seeped in. Those represented about 1–3% of the core volume, according to a paper from Kelemen and others.
In comparison, the mountain where the rock had reacted likely had been exposed to water and CO2 while buried deeply in the ground where geologic testing showed it was 250°C, according to the Scientific American article.
The observations made over the years suggested two things to Kelemen.
- The high heat and pressure on deeply buried rock accelerated the rate of reactions to allow mass conversion.
- Those reactions did something to open the unexposed rock. It is not something he calls fracturing, but there is a common link.
The chemical reactions, such as CO2 and magnesium creating magnesite (MgCO3), expand the volume of the rock downhole creating stress that results in “reaction-driven cracking.” In other words, rather than using hydraulic force, the rock is broken by the stress caused by chemical reactions expanding the rock on a mass scale.
The challenge for 44.01 is to find a way to accelerate the reactions to create enough cracking to expose a large enough volume to mineralize millions of tons of carbon a year. Unlike a one-time event like hydraulic fracturing, a continued high reaction rate could continue opening up more rock to reactions.
A past paper where Kelemen was the lead author found that high temperatures were associated with the high reaction rates, and another concluded that even water with only a “mildly elevated concentration” of CO2 could massively accelerate the reaction rate.
But another paper warns against relying on models based on the available data because “few comparisons of carbon mineralization kinetics exist as a function of temperature, CO2 partial pressure, and other variables.”
Papers have said reaction rates can be accelerated at temperatures ranging from 100 to 250°C (212 to 482°F), with one reference putting the ideal at 185°C (365°F).
The results of lab tests trying to show that that higher temperature and pressures can generate the sustained high rate of reactions needed to sustain chemical reaction-based cracking are mixed. Some suggest it works and others say the growth caused by the process may choke off the flow of the water and CO2 needed to accelerate it.
But, as has been the case in shale, it is hard to replicate in the lab what goes on in the ground. For one, the opportunities to learn by drilling have been limited in hard rock. Field testing is essential to understanding the workings of an unconventional process.
Things To Do
For this test, 44.01 is trying out two solutions for pressing issues on the surface—switching to seawater for injection and testing equipment to capture CO2 from the air.
It began building the Fujairah site in February, which is billed as the first carbon-negative project by an energy company in the region.
It is partnering with ADNOC, the Fujairah Natural Resources Corporation, which manages resources in the emirate with the test site, and ADNOC’s future energies company, Masdar, which will provide solar power.
What they are doing in the ground is similar to the first two pilots in Oman. A relatively small amount of CO2 will be mixed with water and injected 500 to 1000 m deep. A monitoring well nearby will collect fluid samples to measure the volume of water and the CO2 level to see how much of the gas is being mineralized.
It will also be the first peridotite test with injection of seawater. Shifting from fresh water to seawater in this arid land is an essential change. They will need to see the effects of the seawater’s ions and minerals on the process.
Carbon dioxide will be supplied by direct air capture equipment on site. It will be the first use of the that equipment in the extreme heat and humidity of the UAE summer. They want to see how high concentrations of CO2 in the fluid affect the amount of CO2 mineralized. The company would not say how much CO2 will be in the water.
At this stage of testing, direct air capture is the best source of CO2, but their long-term goal is to ramp up for large-scale disposal, likely for captured industrially produced carbon from the UAE and other countries.
Cracking or Clogging
44.01 is planning future tests that will go deeper and inject more water and CO2. Those could help show whether injection into hotter, higher-pressure horizons will massively accelerate the chemical reactions.
For the driller, it will also test the ability to drill an affordable well in hard, hot rock where the ideal temperature for maximizing the mineralization rate may require downhole tools specially built to operate at those extreme temperatures, adding to the cost of drilling.
Those pilots will help address a big question: Will high-volume injection at a depth where the heat and pressure are expected to massively accelerate the chemical reaction rate lead to cracking or clogging?
Lab work can be divided between papers predicting that the level of reactions will be high enough to induce the stress needed for reaction-related cracking, and those suggesting the reactions will shrink already limited flow paths and coat rocks, isolating potentially reactive rock.
But an author of a report whose results would fall on the skeptical side still saw the problems as solvable. Reinier van Noort is the lead author of the paper based on lab work at Utrecht University (UU).
On one side are researchers such as those testing at the University of Utrecht where they injected highly carbonated water through pressurized samples of the various reactive minerals in a peridotite. In four of the five samples tested between 2008 and 2011, they did not observe the expected rock expansion and stress that could lead to cracking.
Instead, the Shell-funded study observed that carbonate rock produced by the reactions filled spaces between the grains of reactive rock used in the test, slowing the reactions to the point where the growth wasn’t sufficient to cause cracking.
“On the whole, we concluded that a force of crystallization could develop during the in-situ carbonation of olivine, but that this process would likely become transport-limited and, thus, slow down to rather low rates,” van Noort said.
The paper said that using “rigorous but simple estimates based on the experimental results, we conclude that the in-situ mineralization of CO2 in peridotite bodies may take 300–6,000 years to proceed by one meter and is thus likely to be too slow.”
Since that work was done more than a decade ago, van Noort has become a researcher with the department of reservoir technology at the Institute for Energy Technology (IFE) in Kjeller, Norway, where he is working on a different use for reactive rock as a key ingredient in self-healing cement, including minerals that expand when they react with fluids in oil wells. While he is focusing now on creating clogging, he is hopeful that reaction-driven cracking can be made to work in spite of it.
“While the work I did at UU has shown that it may not be as easy as others claim (or claimed, before our work came out), I do believe that it will be possible to find ways around the inhibiting effects we reported; but I think it might require more lab work (and perhaps some process engineers) to get things going,” wrote van Noort in an email. He added that an IFE colleague received funding to set up a research project on “in-situ mineralization in mafic rocks,” which include peridotites.
One possibility is finding a way to separate the rock dissolution by water and the carbonate-precipitation steps “so that flow pathways through the peridotite rock can be maintained, or even widened, due to dissolution, while precipitation takes place where space is not as limited.”
Kelemen has said that other test results have indicated that accelerated reactions do not have negative effects, in part due to reaction-driven fracturing, but more field testing is needed to understand these little-known formations.
“It is essential to understand the chemico-physical parameters that lead to cracking vs. clogging, which can then be applied to favor cracking in reservoirs and clogging in caprocks,” Kelemen wrote in the 2019 paper. “Currently, the size, injectivity, permeability, geomechanics, and microstructure of peridotite reservoirs are relatively unknown. Understanding nano-scale rock behavior is essential to understanding the macro-evolution of the reservoir, and more research is needed in this field. In order to assess the possibility of CO2 storage in peridotite formations, two to three small-to medium-scale pilot projects are needed. This will lead to a greater understanding of the behavior of hierarchical fracture networks, which are difficult to simulate in laboratories.”
For Further Reading
Rare Mantle Rocks in Oman Could Sequester Massive Amounts of CO2 by Douglas Fox, Scientific American.
With Major Prize, a Project to Turn Carbon Emissions to Stone Gains Momentum by Kevin Krajick, Columbia Climate School, Columbia University.
Vein Distribution in Ultramafic Basement of the Wadi Lawayni Drill Sites by P.B. Kelemen, Columbia University; W. Bach, University of Bremen (Germany); and A. Eslami, University of Tehran (Iran), et al. American Geophysical Union, Fall Meeting 2018.
In-Situ Carbon Mineralization in Ultramafic Rocks: Natural Processes and Possible Engineered Methods by P.B. Kelemen, Columbia University; R. Aines, Lawrence Livermore National Lab; and E. Bennett, Cardiff University, et al.
An Overview of the Status and Challenges of CO2 Storage in Minerals and Geological Formations by P. Kelemen, Columbia University; S.M. Benson, Stanford University; and Hélène Pilorgé, Worcester (Massachusetts) Polytechnic Institute, et al.
The Force of Crystallization and Fracture Propagation During In-Situ Carbonation of Peridotite by R. van Noort, Utrecht University; T.K.T. Wolterbeek, Institute for Energy Technology (Norway); and M.R. Drury, Utrecht University, et al.