Present industry solutions to the challenge of well spacing involve expensive geomechanical Earth modeling or fracture-geometry monitoring that is time-consuming, data-intensive, and geography-specific. The authors of the complete paper write that their developed methodology is quicker and pad-specific and requires no additional data collection than those routinely gathered. Other significant outcomes of this work flow include the ability to rank well performance and predict changes in geology and pressure regimes relative to an existing similarly landed producer.
Introduction
Despite more than a decade of the shale revolution in the US, present well-spacing techniques are far from ideal. Given the spatial variation of reservoir properties, this problem becomes localized or pad-specific.
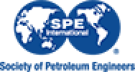