Pseudo dry-gas (PDG) technology has been demonstrated successfully for transporting wet gas in a 200-km subsea tieback pipeline in deep water under wet-gas conditions (water-saturated gas). The technology helps reduce the inlet pressure by reducing the liquid content and, therefore, the gravitational pressure drop. The mitigation of the gravitational pressure drop allows the use of larger pipelines to minimize frictional pressure drop, which in turn increases reserves recovery and allows tieback distances to be enhanced.
This paper investigates a pseudo dry-gas system for an ultralong deepwater gas condensate development, building upon research and development already conducted with Strathclyde University. This work was undertaken using nonstandard flow assurance methodologies and simulations, recycling data and results with the advanced computational fluid dynamics simulations of the liquid-removal units’ behavior.
Introduction and Background
Successfully transporting wet gas over long distances must overcome numerous issues, including high back pressure and slugging based on standard gas transportation systems. These issues historically limited the length of tiebacks to 110 km for a single pipeline and 150 km for dual pipelines.
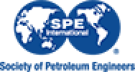