Monitoring the waterflooding oil-recovery process is a difficult task for seismic-based methods in hard carbonate reservoirs. The changes in velocity and density caused by water/oil substitution are too small when compared with the errors involved in repeating the measurements. The authors detail the development of a technique based on surface-to-borehole controlled-source electromagnetics (CSEM), which exploits the large contrast in resistivity between injected water and oil to derive 3D resistivity distributions, proportional to saturations, in the reservoir.
Introduction
CSEM techniques for reservoir-fluid characterization and monitoring are applied on a commercial basis for cross-well configurations. The method is based on electromagnetic (EM) induction and, as such, uses magnetic sources and magnetic receivers.
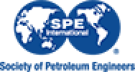