Large amounts of clay minerals are present in shale; shale’s resulting instability is a major problem while drilling. This paper introduces a novel idea to improve shale stability by using low-temperature drilling fluid and cost-effective thermoelectric and nickel-iron (Ni-Fe) nanomaterials to alter the mud temperature. The thermoelectric nanomaterial reduces the mud temperature as electricity is applied, while the Ni-Fe nanomaterial helps to increase the mud temperature.
Introduction
Shale instability is a result of several factors. (For a detailed discussion of these factors, as well as three models used to determine stresses and to calculate formation pore volume, please see the complete paper.) Shale instability has considerable negative effects on the cost and speed of drilling operations. Three factors that predominantly affect the shale stability are chemical, thermal, and hydraulic effects. Because of these factors, a change in pore pressure (and thus a change in the stress on the wellbore) is experienced. Improved shale stability can be achieved by minimizing the effect of these factors. Chemical effects can be minimized by upholding the salt concentration in drilling mud, but countering thermal effects can be a formidable task.
This paper discusses a novel process to miniaturize the thermal factor influencing shale stability. The thermoelectric nanomaterial is specially used to cool down the mud temperature. The second nanomaterial used exhibits self-heating properties and thus increases the temperature of mud. The nanomaterials are used in a way that depends upon the respective conditions needed to maintain the optimum temperature difference between the formation and the drilling fluid.
Rock Failure
Wellbore instability comprises compressive failure, hydraulic fracturing, and radial spalling. Compressive failure is observed when the heating of formation takes place (i.e., the mud temperature is greater than the formation temperature). Because of the heating process, expansion of shale takes place, thereby promoting compressive failure. Compressive failure increases the chances of pipe sticking. This compressive failure is determined by the compressive shear failure potential.
Hydraulic fracturing is the failure that occurs when fracture inside the formation takes place along the radial direction. This occurs when the temperature of the mud is lower than that of the formation. Lost circulation is observed because of hydraulic fracturing. Hydraulic-fracturing failure is determined by the hydraulic-fracturing potential. This hydraulic-fracturing-potential value is equal to the tensile stress.
Radial spalling is the failure that occurs when fracture inside the formation is perpendicular to the radial direction. Pipe-sticking and hole-enlargement problems are observed because of radial spalling. This radial spalling is determined by the radial-spalling potential. When the radial-spalling potential is less than 0, radial spalling occurs.
Experimental Test of Thermal Effects
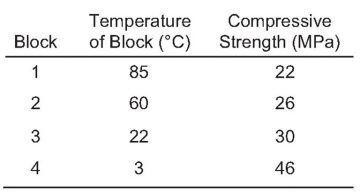
The main objectives of experimentation are to observe the variation in strength with changing temperature conditions and to examine the potential for application of low-temperature fluid.
Experimental Setup. The experimental setup consists of a material-testing machine with a built-in load frame, force transducers, movable cross heads, and extensometers. The placement of the block is made in such a way that one face is open to the atmospheric temperature (22°C) and the other remaining faces are placed according to the temperatures mentioned in Table 1.
Summary of Test Procedure. The four blocks are placed in this temperature system for 12 hours; then, the cement blocks are strength tested on a universal testing machine. This machine measures the compressive strength of a cement block. The compressive strength of these blocks is shown in Table 1, and the results obtained are plotted in Fig. 1.
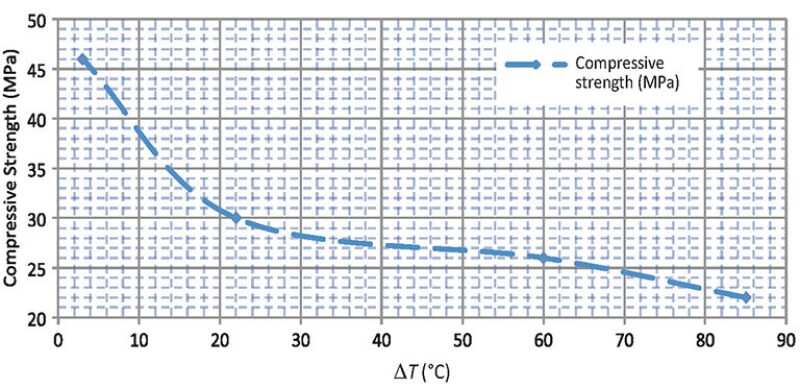
Nanomaterial
As mentioned previously, rock failure caused by high-temperature mud increases the severity of compressive, radial-spalling, and hydraulic-fracturing failure. Similarly, for very low temperatures, tension occurs, resulting in problems such as hydraulic fracturing and radial spalling. It is therefore essential to control the temperature difference between the mud and the formation.
To control the temperature of drilling mud, this paper suggests the use of two types of nanomaterial. These are thermoelectric [bismuth telluride (Bi2Te3)] and Ni-Fe nanomaterials. The thermoelectric nanomaterial is used to decrease the temperature of the drilling fluid, whereas the Ni-Fe nanomaterial is used to increase the temperature of the drilling fluid.
Thermoelectric Nanomaterial. Recently, significant research has been carried out on thermoelectric nanomaterials to increase their efficiency and to explore new versions. These nanomaterials are used typically as coolants in motorcycle and car engines. They are also used to convert the waste heat energy to a much more usable electric energy. These nanomaterials offer advantages such as reduced pollution and noise, increased reliability owing to a lack of moving parts, and cost effectiveness. The basic mechanism of this nanomaterial is based on the thermoelectric phenomenon. (For a discussion of the mathematical bases of thermoelectric effects such as the Seeback effect and the Peltier effect, please see the complete paper.)
Various thermoelectric nanomaterials are used for the cooling process. The main comparative factor for these nanomaterials is their figure of merit, which varies with temperature. In this paper, the authors have used the Bi2Te3 superlattice thermoelectric nanomaterial.
For current drilling conditions, Bi2Te3 is a most suitable nanomaterial, and its figure of merit is good for low-temperature conditions. The figure of merit of the Bi2Te3 superlattice thermoelectric material lies between 2 and 3. As the figure of merit increases, the coefficient of performance also increases.
The temperature drop is increased by increasing the total current applied at the junction. As the current flow is increased, the temperature drop increases by 25°C. The temperature drop also depends on the temperature of the hot junction. For a hotter-junction system, the temperature drop will be greater.
Equipment. The heat exchangers used currently for cooling tend to be bulky. The advantages of the application of nanomaterial in cooling have been mentioned previously, but it is also important to make the equipment size smaller as compared with currently used heat exchangers.
Here, we use the tubing with layers of (a) asbestos, (b) Bi2Te3 nanomaterial, (c) an insulator, and (d) a steel cover. These are layered from inside to outside, as shown in Fig. 2 above.
The asbestos layer is put immediately next to the mud-conducting tubing to prevent contact between the Bi2Te3 layer and the mud-conducting tubing. It also allows heat to transfer.
The Bi2Te3 nanomaterial layer is placed next to the asbestos layer. The application of this Bi2Te3 layer is intended to decrease the temperature of mud when the current is applied across it. The insulator layer above the Bi2Te3 layer is used to prevent the flow of current outside, and heat flow inside, the tubing. This should be a good insulator of current and heat. The steel cover is used above the insulator layer to give firm support and give protection to all layers from any externally induced damage.
For good cooling results, this equipment is atomized. For this, four temperature indicators are placed at equal distances along the mud-circulating tubing. This divides the tubing into three sections; these indicator signals are transferred to the current-controlling device that controls the current applied to the different sections of tubing. This eventually controls the final temperature. The first indicator is used to measure the initial temperature of mud entering the equipment.
On the basis of the noted temperature in the first indicator, the current is controlled for the first section of tubing. A similar process is carried out for the second and third indicators in the second and third sections, respectively.
This atomization is important because the mud never reaches a constant temperature at the wellbore because of different processes carried out while drilling. For example, while drilling, the temperature of mud at the outlet will be high, whereas during circulation, the mud temperature at the outlet will be low.
Ni-Fe Nanomaterial. In some extreme environmental conditions, such as in the Antarctic and in deep water, very-low-temperature environments are encountered. As mentioned previously, for very-low-temperature mud (∆T=50°C), problems such as hydraulic fracturing and radial spalling increase. Therefore, in such an environment, there is a requirement to increase the temperature of mud. For this purpose, the Ni-Fe nanomaterial is used. This nanomaterial has self-heating properties, and when it is mixed in mud and the mixture passes through a magnetic field, mud temperature increases.
The bases of this nanomaterial are hysteresis and relaxation losses under the magnetic field. Therefore, when an alternating magnetic field is applied on this nanomaterial, because of the aforementioned losses, heat is dissipated. This dissipated heat increases the temperature of mud.
To increase the temperature of mud, the Ni-Fe nanomaterial is first mixed in the mud. When this mud is passed through the magnetic field, the heat is dissipated, which directly increases the temperature of the mud (this nanomaterial can increase the temperature of mud by 42°C).
This increase in temperature depends on the magnetic flux applied. For high magnetic flux, the increase in temperature will be high.
Equipment. This equipment is the same as the cooling equipment. The only difference is that above the tubing, there is an electromagnet that produces the magnetic flux by which the heat is dissipated. In this electromagnet, a soft iron block is present that is surrounded by a copper coil. When current flows through the copper coil, a magnetic field is generated. Atomization is also important in this equipment to prevent overheating.
This article, written by JPT Technology Editor Chris Carpenter, contains highlights of paper SPE 164000, “Thermoelectric Nanomaterial: A Demiurgic Approach Toward Improvement of Shale Stability,” by Prateek Pandey, SPE, Pratik Kapadnis, SPE, Bilal Ghansar, SPE, and Sagar Mandan, SPE, Maharashtra Institute of Technology (Pune), prepared for the 2013 SPE Middle East Unconventional Gas Conference and Exhibition, Muscat, Oman, 28–30 January. The paper has not been peer reviewed.