An 18-well numerical-simulation model was built to represent an operator’s Lower Shaunavon waterflood-pilot area. Numerical simulation was used, and a history match on the pilot area was performed. By use of parameters obtained from the history match, a representative model was built and a sensitivity study was performed on hydraulic-fracture spacing and well spacing in both primary-depletion and waterflood scenarios.
Modeling-Work-Flow Description
The Shaunavon is partitioned into lower and upper members. The lower member is an authigenic carbonate shelf, while the upper member has a strong clastic influence from the west.
×
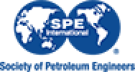
Continue Reading with SPE Membership
SPE Members: Please sign in at the top of the page for access to this member-exclusive content. If you are not a member and you find JPT content valuable, we encourage you to become a part of the SPE member community to gain full access.