Gas-injection huff ’n’ puff enhanced-oil-recovery (EOR) techniques have the potential to improve liquid hydrocarbon recovery in ultratight, unconventional reservoirs. This paper studies the technical and economic viability of this EOR technique in Eagle Ford shale reservoirs using natural gas injection, generally after some period of primary depletion, typically through long, hydraulically fractured horizontal-reach wells.
Reservoir-Analysis Work Flow
Model Description. A compositional, fine-scale, dual-porosity, dual-permeability, symmetry-element numerical model was used in this study to model the current primary depletion and the EOR huff ’n’ puff process. In this study, the element of symmetry, which represents the bottom half of a cluster within a fracturing stage, is being extended to include three wells to allow the investigation of the effect of interference and containment on pad-level cyclic-gas-injection deployment.
Production and injection of the entire well come from all active stages in each well, with equal weighting. The production and the injection for each cluster in every well are calculated proportionally by use of these assumptions.
Model Input. The complete paper describes the critical input for the numerical model, including fracture half-length and fracture height, shape factor, absolute and relative permeability, and pressure/volume/temperature (PVT).
History-Matching Work Flow. Because the history-matching solution is known to be highly nonunique, a comprehensive probabilistic approach was used to identify the realistic solution space.
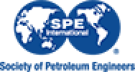