Geothermal energy is “having a moment.” It has been part of the global energy mix for many decades, but growth has been limited by the relatively limited number of sites with optimal geologic conditions. Technologies developed in the oil and gas sector have the potential to overcome these limitations and unlock dramatically increased geothermal production in the US and worldwide. Success is far from certain, but with the enthusiasm, ingenuity, and capital flowing toward these technologies, there is a realistic shot at breakthrough success.
Geothermal energy comes in a variety of flavors, from shallow heat-pump systems used for residential heating and cooling to deep, hot wells drilled for electricity production. While opportunities exist across this spectrum, this column is specifically concerned with the drilling of deep, high-temperature wells for electricity production.
Flow rate is a major challenge for geothermal. The energy content of one barrel of hot water is much lower than the energy content of one barrel of oil. Thus, to achieve profitability, geothermal wells must produce the equivalent of tens of thousands of barrels of water or steam per day. Across much of the western US, temperatures are hot enough for geothermal electricity production within reasonable drilling depth. However, in the absence of special geologic conditions from a hydrothermal system, wells usually cannot produce sufficient rate.
Dating back to the 1970s, hydraulic stimulation has been tested as a way of increasing flow rate per well. Designs utilize injection and producer wells, with fluid heating up as it circulates between the wells. If engineers could use stimulation to consistently achieve high flow rates, a vast resource would be unlocked. The US Department of Energy’s (DOE) 2019 GeoVision report estimates that more than 60 GWe could be produced in the US by 2050.
To date, success with hydraulic stimulation has been limited. Conventionally, geothermal wells have been drilled vertically and then stimulated by injecting only water (no proppant) into a single openhole section (without using multiple stages). Engineers have hoped that the injected water would shear-stimulate a dense network of natural fractures, with a large amount of surface area and fracture conductivity. These designs can yield modest success because geothermal wells are usually drilled in high-strength rock in the crystalline basement, and so fractures have considerable self-propping capability. However, rather than creating a dense network, flow tends to localize into a small number of dominant flowing pathways. Without a large number of flowing pathways, the reservoirs lack the ability to sustain high rate and are subject to thermal short circuiting that prematurely reduces the production temperature.
Fortunately, similar problems have been encountered and solved by the shale industry. The shale revolution was unlocked by the application of multistage hydraulic fracturing along horizontal wellbores. Mechanical isolation allows sequential injection into sections of the well. Within each stage, perforation pressure drop is used to force fluid to flow into multiple pathways along the well. Perforation clusters are spaced tightly—typically from 10 to 50 ft. Recent core-through studies confirm that stimulation is creating hundreds or thousands of conductive fractures along each fractured lateral.
Early shale designs used widely spaced perforation clusters or openhole swellable packer systems and used relatively small volumes of proppant. Analogous to the traditional thinking from the geothermal industry, there was a hope that the water injection would naturally create a dense, well-connected fracture network.
But over the past decade, field experience and diagnostics have shifted the industry’s thinking. Core-through studies and offset fiber show consistently oriented hydraulic fractures propagating in relatively narrow bands. Production results have led to the abandonment of openhole designs in favor of limited-entry designs. Rather than hoping that a complex fracture network will form naturally from injection, engineers use technologies to distribute flow and force a huge number of fractures to form.
The techniques used in shale to prevent flow localization can be applied directly to geothermal. This is a big deal. If we can create hundreds or thousands of flowing fracture pathways around a horizontal or deviated geothermal well, then we will have truly changed the game.
For geothermal to reach its full potential, there will need to be many other improvements and a relentless focus on reducing cost. We need better drilling technologies, high-temperature downhole tools, reservoir engineering and modeling, and much more. If companies can demonstrate success in generating sufficient flow rate and heat-exchange area, this will justify the investment of capital to improve the supporting technologies.
In the near future, multistage hydraulic stimulation for geothermal will be put to the test. The DOE FORGE project in Utah is pursuing multistage stimulation in highly deviated wells in granitic rock. Fervo Energy will soon be piloting commercial-scale multistage geothermal systems. The DEEP project in Canada recently executed a multistage stimulation for geothermal production in a sedimentary formation.
What Are the Risks?
First, these designs depend on achieving adequate fracture conductivity. Circulating fluid through hundreds—perhaps thousands—of fractures will mitigate that risk, but nevertheless, until these systems have been tested, we will not have good field-scale data on our ability to sustain good long-term fracture conductivity. Proppant should be tested to enhance conductivity. Second, as the rock cools down, it shrinks, which decreases stress and increases fracture conductivity. This has potential to create feedback loops that cause short-circuit paths to develop between the injector/producer wells. Third, high temperatures make everything more difficult operationally. The industry needs to develop more robust high-temperature downhole tools and needs to improve the reliability of the large-diameter plugs required for mechanical isolation in geothermal wells.
Induced seismicity is also a key issue. Fluid injection can cause slip on faults. There have been several instances of geothermal projects cancelled by induced seismicity that was felt at the surface. The DOE organized the development of a best practices induced-seismicity protocol that lays out a systematic process for addressing induced seismicity, including the use of a “traffic light” system. It is critically important for geothermal projects using hydraulic stimulation to follow this protocol’s best practices. If they do, then this technology can be used safely and effectively.
In my opinion, the surest path to success for geothermal will be to adapt the technologies that have been wildly successful in shale. Bold entrepreneurs, scientists, and engineers are turning these ideas into field pilots, and if they are successful, they will have a tremendous positive impact.
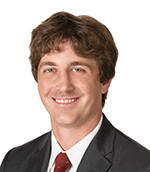
Mark McClure established ResFrac in 2015 to help operators maximize value through the application of advanced geomechanics and reservoir simulation. Before founding ResFrac, he was an assistant professor at The University of Texas at Austin in the Department of Petroleum and Geosystems Engineering. He holds a BS degree in chemical engineering, an MS degree in petroleum engineering, and a PhD in energy resources engineering, all from Stanford University. His academic research focuses on hydraulic fracturing, diagnostic fracture injection tests, induced seismicity, and enhanced geothermal systems.