Increasing sensitivity to oil price fluctuation requires operators to reduce costs of operation. A key component is collaborative management by operators and suppliers to reduce cost over the full life cycle of the operation. Much of the focus is on the equipment-purchasing strategy; nonetheless, effective technology such as an autonomous inflow control valve could also benefit the operating expenditure (OPEX) savings by enhancing oil recovery and reducing processing costs of excessive unwanted water and gas in surface facilities.
OPEX comprises labor, chemicals, consumables, infrastructure maintenance, transportation, disposal, and other utilities. Capital expenditure (CAPEX) may also be reduced for long-term projects, which generally comprise infrastructure such as pipelines, pumps, disposal wells, ponds/storage treatment facilities, and other associated utilities infrastructure.
The average cost for handling produced water starts from about $1.50/bbl, depending on the geographical location of the operation. Such costs may justify an assessment of an autonomous inflow control valve (AICV) to reduce unwanted water and gas to achieve OPEX savings.
Horizontal wells are a proven option to maximize reservoir contact in certain situations. A challenge is often early water breakthrough in less than 6 months from starting production, and then progressively producing very-high water cuts, often 95%, for long periods of times thereafter. Inflow control device (ICD) technology has demonstrated potential in improving recovery in horizontal oil wells by delaying the gas and/or water breakthrough. However, ICDs cannot restrict water effluents once breakthrough occurs. A new generation of inflow control technology known as an AICV can balance the production flow and choke and shut the water-effluent zones once breakthrough occurs.
Basis of Design
The design is based on the Hagen-Poiseuille equation and Bernoulli’s principle. It uses the difference in fluid-flow behavior in the laminar- and the turbulent-flow restrictor to differentiate the pressure drop using the mobility ratio between oil, water, and gas. Light-viscosity fluids such as water and gas have high mobility and allow the AICV to detect the mobility difference, then choke and shut the main inflow area in the valve autonomously. Fig. 1 illustrates the construction of the valve in open positions, showing the direction of flow with arrows and the P1, P2, and P3 pressure locations. The inlet pressure is represented by P1: 2–5% of the main flow will move through the laminar-flow element (LFE) that will reduce the pressure to P2. Further pressure reduction occurs in the turbulent-flow element (TFE) to P3 as the tubing pressure. The differential pressure in LFE reacts to the piston’s effective area, resulting in a force balance around the piston to control the valve’s function (e.g., to open, choke, or close the main flow).
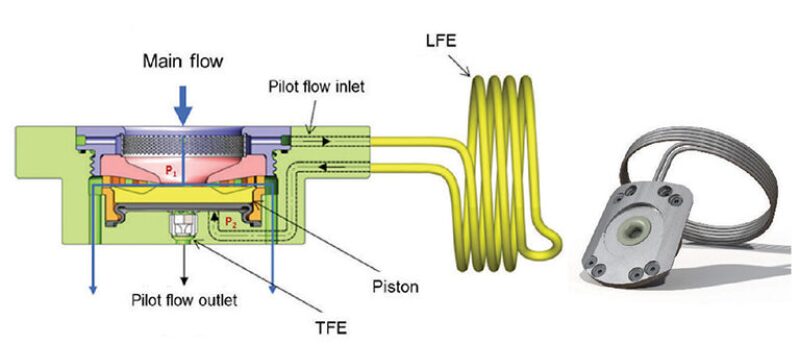
The design was tested in single-phase and multiphase flow loops that replicate the downhole operating conditions. Fig. 2 shows the results of a laboratory experiment of single-phase AICV (solid line) flow performance for 2.7 cP oil, gas, and water with a corresponding regular nozzle ICD (dashed line). The plotted data represent the pressure drop across the device as a function of flow rate for a single device. AICVs have demonstrated more than a 90% improved choke of water (blue) and gas (red) compared to ICD but retain the oil (green) performance.
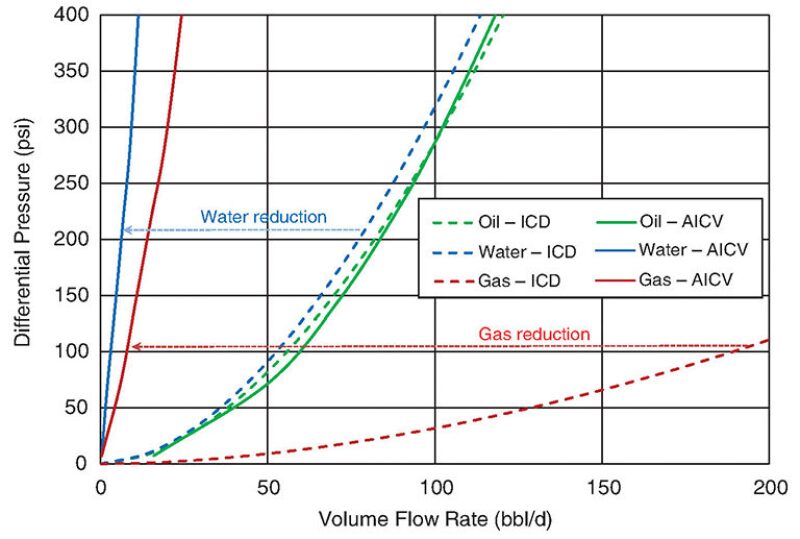
Case Study: Reduced Water Cut
A Middle East oil field producing for several years encountered water control issues. The reservoir is a sandstone, mature waterflood play. Newly drilled wells typically started their production life with 60–80% water cut and quickly increased to 80–90% within the first 6–8 months of production. The operator had issues managing the high water production volumes; significant oil remained bypassed/stranded. Choking the well back at surface required extra expenditure for treatment facilities capable of handling large volumes of produced water.
The operator applied AICV in lower well completions for more than 10 wells. By reducing the high water cut, the reservoir pressure was enhanced, and the wells produced less water. The average oil production increased by 18–20% and water cut was reduced by 68% on average. OPEX savings of $240,000 were realized within the first year in the operation of the water-handling facilities at surface. Fig. 3 highlights data from six wells using AICV.
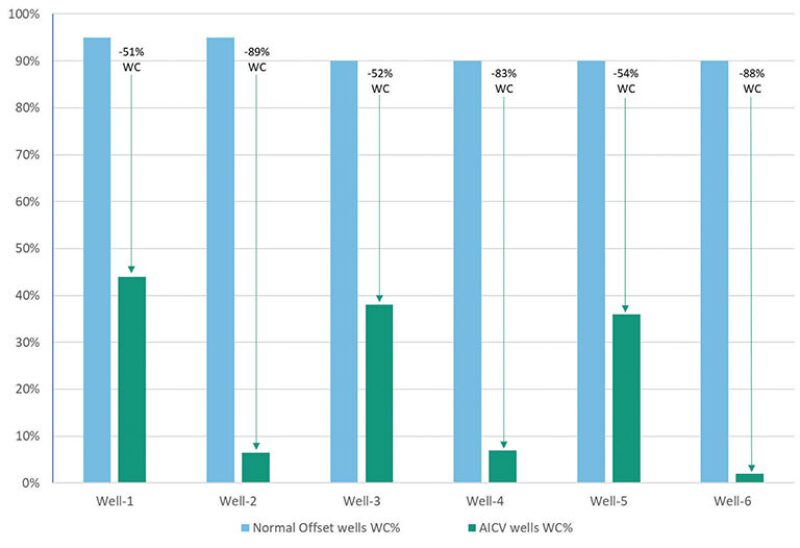
Case Study: Reduced Gas Production
A Middle East operator produced oil from a field with a gas cap since the 1960s. It has strong gas communication throughout its fracture network. Gas breakthrough occurred throughout the field, and many wells also suffered from a large increase in water production. Without downhole control mechanisms, the operator choked the wells back; in more severe cases, the high gas/oil ratio (GOR) made the wells unproducible, resulting in shut-in wells. Significant oil reserves and production remained stranded.
The operator applied AICVs in lower well completions for over 20 wells to manage the unwanted gas and water. The average oil production increased by more than 56%, gas production decreased by 80%, and the water cut was reduced from 80 to 26%. The reduction in water and gas production enabled a stranded, shut-in well to become economic and resulted in OPEX savings of over $200,000 within the first year.
Case Study: Gas Reduction in Ultralight Oil Retrofit
A carbonate oil field in the Middle East had significant gas communication through its fracture network. Gas breakthrough and a high GOR made the well unproducible. The reservoir oil viscosity is between 0.4 and 0.6 cP in contrast to gas at 0.02 cP. The operator shut in wells, resulting in stranded oil reserves and production. The reservoir, log, and production data were studied to design the well completion.
The operator applied AICV lower-well completions to manage the gas and allow oil production. Shutting in high-GOR zones with the valve redistributed the tubular drawdown to the oil-saturated zones, leading to increased oil production and total oil recovery from the reservoir.
Within the first 12 months of the well having been brought on line with the AICV retrofit completion, the operator earned a net gain of over 51 times the cost of the retrofit completion, including rig costs. The well has been stable for over 30 months at the time of this publication.
The AICVs increased the average oil production by more than five times from the lower well section and enabled the entire wellbore to contribute to oil recovery and reduced gas by 85%. The production logging (PLT) results of cumulative oil and gas along the well before and after installing the AICV are available in IPTC 20195.
Case Study: Heavy-Oil Water Control
A sandstone oil field in western Siberia with an oil-saturated rim, an extensive gas cap, and underlying water is characterized by weakly consolidated sandstone and high-viscosity oil. The operator expected significant early water and gas breakthrough into the wellbore. The complex trajectory of long horizontal wells up to 2000 m and the presence of highly permeable channels, tectonic faults, and thin oil rim made the development of the project challenging.
Field experience indicated that the well could not effectively be operated without a flow control device. Various types of autonomous and active flow control devices were applied during the technology trial campaign and Phase 1 development.
The data in Fig. 4 show the following (SPE 196851):
- AICVs produce 70–80% less water vs. AICD
- AICVs produce 20–30% more oil vs. AICD
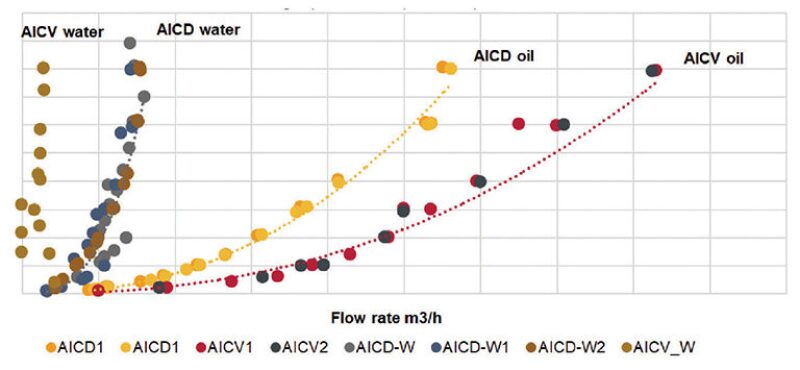
References
Case Study: Utilizing Autonomous Inflow Control Valves Helps To Have Better Fahud Wells Production Performance. H. Maamari, PDO; M Abd El-Fattah and V. Mathiesen, InflowControl AS. Mediterranean Offshore Conference and Exhibition, Alexandria, Egypt, 15–17 October 2019.
OTC 28860 Autonomous Inflow Control for Reduced Water Cut and/or Gas/Oil Ratio. A. Elverhoy, H. Aakre, and V. Mathiesen, InflowControl AS. Offshore Technology Conference, Houston, Texas, USA, 30 April–3 May 2018.
IPTC 20195 Gas Production Optimization Using AICV Technology. Z. Alali and A. Musharraf, Saudi Aramco; M. Abd El-Fattah and V. Mathiesen, InflowControl AS. International Petroleum Technology Conference, Dhahran, Saudi Arabia, 13–15 January 2020.
SPE 196851 Enhancing Efficiency of High-Viscosity Oil Development With Autonomous Flow Control Device. Case Study in Western Siberia. T. Solovyev, Sevkomneftegaz. SPE Russian Petroleum Technology Conference, Moscow, Russia, 22–24 October 2019.
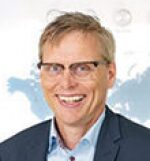
Vidar Mathiesen is CEO and cofounder of InflowControl AS. He has been head of technical research support at Statoil’s research center, and has extensive experience in innovation and product development, qualification, modeling, installation, and evaluation of ICD/AICD as a leading research scientist at Statoil and Hydro. He has written several articles published in international journals and conferences, submitted several patents, and received various innovation awards. Mathiesen and Haavard Aakre are also the inventors of the Statoil-AICD, the RCP.
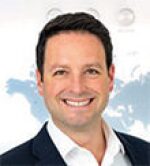
Brent Brough is CCO of InflowControl AS and has over 18 years of international experience, including managing and leading diverse global teams in Canada, Russia, UK, Norway, and UAE. He helped build large and small technology companies that delivered growth through their technology. Brough graduated from Southern Alberta Institute of Technology in chemical engineering and holds an MBA from Warwick Business School in Global Energy.