As operators move into more remote regions, deeper offshore depths, and into regions that yield more challenging reservoir fluids, they face difficulty in keeping the produced fluids and gas flowing through the lines. The field of flow assurance, the coupling of multiphase flow and production chemistry, was born out of necessity as it has grown in concern to all operators.
To effectively manage flow assurance, oil and gas operators must take a holistic approach, particularly when working in deepwater offshore environments, Abul Jamaluddin, NExT business manager for North America and a flow assurance adviser to Schlumberger said. Speaking during a recent SPE training course webinar on flow assurance, he said that the best approach was one which considered all aspects of the problem.
Flow assurance is essential to the economics of any project and the overall goal is to maintain flow from wellbore to processing. “Flow assurance is a problem that has always been around in our industry,” he said. But the problem has grown more complex as operators move into more challenging environments. In shallow water or onshore, operators often got a lot of solids deposits in the production system, but gaining access to the wellbore and the flowlines was easier. In deep water, operators have to contend with colder temperatures, higher pressures, and longer tiebacks. Deepwater wellbores are more expensive to access and present more complexity in mitigating blockage of flowlines. “(In deep water) you have basically one chance to drill the producing well and the ability to understand the reservoir fluid characteristics because these wells are very expensive,” Jamaluddin said.
Flow assurance depends on a variety of factors, including reservoir fluid properties, fluid flow, asphaltene, wax, hydrates, naphthenates, scales, heavy oils, emulsions, foams, sand, and heavy oils. “Understanding the fundamentals of these elements is the key to designing a management strategy,” he said. To address these concerns, operators must work in three domains when maintaining flow assurance: production chemistry, production engineering, and production surveillance.
Jamaluddin urges operators to start with formation testing technologies to determine the nature of the reservoir’s fluids. “We want to know what kind of fluid we are dealing with in terms of API, gas/oil ratio, and compositions,” he said. Representative samples are taken from the reservoir for a series of laboratory tests, including pressure, volume, temperature, asphaltenes, waxes, and hydrates—measurements that lead to effective modeling of the reservoir.
Operators need a system in place for monitoring field production to ensure that the production conforms to the model’s predictions. Finally, a feedback loop using sensors and multiphase flow meters enables an operator to make changes to the system if the production parameters deviate too far from an initial design.
When planning for flow assurance, operators must bear in mind that reservoirs are not generally homogeneous. “In the past, we used to think of a reservoir as a tank of diesel, with compositions the same throughout,” Jamaluddin said. But many reservoirs have varying compositions, with lighter fluids at the top and heavier fluids at greater depths. “We usually have a mixed fluid with light ends and heavy ends,” he said. The mixture of the two can pose additional challenges, such as the precipitation of the asphaltenes commonly found in heavy fluids. Asphaltenes can precipitate due to changes in oil composition, temperature, or pressure depletion.
Common inorganic scales, such as calcite and barite, also present challenges. Calcites are frequently formed when produced waters from high-bicarbonate and high-calcium zones of the reservoir are commingled.
Barite generally forms when there is a mixture of production and injection water. The barium concentrations in produced water are roughly inversely proportional to the concentration of sulfates, indicating that the barium is in equilibrium with barite in the formation. If saline water is injected into the reservoir and mixes with the formation water, the concentration of sulfate in the production water may cause the super-saturation of barite.
Hydrates form in combinations of high pressure, low temperatures, and low-molecular-weight gases (methane, ethane, propane, or butane). Water molecules arrange themselves into 5- or 6-membered rings, which form three-dimensional polyhedra around the gases. One volume of hydrate can carry from 160 to 180 volumes of methane, which presents unique risks to deepwater offshore operators, such as the abrupt expansion of the methane gas during the breakup of hydrates, Jamaluddin said.
Hydrates Management
Amadeu Sum, associate professor and co-director of the Center for Hydrate Research at the Colorado School of Mines, said that temperatures do not have to be below freezing for hydrates to occur. Seafloor temperatures in the deepwater Gulf of Mexico (GOM) are close to 4°C year round.
Hydrates can form onshore or offshore in a relatively short period of time, plug up lines quickly, and disrupt production. Within flowlines, hydrates can form along the walls or can flow through the production, forming a solid slurry, he said.
A common approach to prevent hydrates from forming in flowlines is to inject thermodynamic inhibitors—such as methanol, monoethylene glycol (MEG), or ethanol—at the wellbore. “Using this approach, it is therefore important to have a good understanding when hydrates can form, in terms of temperature and pressure, in a flowline,” Sum said.
A tool used to determine the correct amount of a hydrate inhibitor is the Colorado School of Mines Gibbs Energy Minimization (CSMGem). It allows a user to enter specific data on a hydrocarbon mixture and obtain a pressure/temperature equilibrium boundary with and without a thermodynamic inhibitor. Methanol has often been shown to be the most effective on a per weight and cost basis, while MEG is more commonly used for gas systems. Ethanol is a predominant inhibitor in Brazil, he said.
Instead of trying to eliminate hydrates entirely, which incurs additional operating expenses for chemicals, operators are considering alternative approaches. One of these is to allow hydrates to form, while working to prevent their agglomeration. The strategy allows operators to tolerate some hydrates in flowlines without blocking the lines, and to reduce the cost and quantity of chemicals used, Sum said.
Although less expensive, there is additional risk to this approach: Once hydrates start to form, they can quickly plug up lines and force operations to a halt. The key to hydrate management is to inject anti-agglomerants, which keep hydrates dispersed in the fluid phases, and thus, prevent them from forming larger agglomerates that can plug the line, he said. For this approach to be successful, one needs to have a good understanding of how hydrates are forming, agglomerating, depositing, and jamming.
The Center for Hydrate Research has noticed trends in hydrate formation. Before the hydrates are formed, the turbulence of the flow emulsifies water in oil and entrains gas bubbles in the liquid. When the temperatures are low enough and pressures are high enough, hydrates will most likely form at the interface between the water and the hydrocarbon fluid, whether oil or gas, forming a hydrate shell around water or oil droplets that are in the emulsion. Hydrates can also form along the pipe walls, which are continuously wet and exposed to the gas.
The amount of water, gas, or temperature variations can affect the growth rate of hydrates. The initial hydrate shell probably forms very quickly. From there, mass or heat transfer affects the growth rates of the hydrate crystals. Once a sufficient amount of hydrates are in the system, a slurry will change the flow behavior as hydrates are either suspended in the oil/water fluid or deposited on the walls of the pipe. The hydrate particulates may attempt to agglomerate into larger aggregates or continue to grow in larger deposits on the pipe wall, depending on the flow conditions of the continuous fluid. If the hydrates are dispersed in a continuous water phase, the hydrates will remain dispersed. If, however, the particles are dispersed in an oil-continuous phase, they will likely build into larger aggregates.
The Colorado School of Mines has developed two tools, CSMHyK (Colorado School of Mines Hydrate Kinetics) and CSMHyFAST (Colorado School of Mines Hydrate Flow Assurance Simulation Tool), to model the formation of hydrates and the resultant plugging in production systems.
Mitigation of Paraffins: A Case Study
Nathan Goodman, operations drilling engineer at Anadarko, said deposition of paraffin and consequent plugging are among the most most pressing issues in deepwater GOM, after hydrates.
One well under study was located in the Power Play prospect, discovered in 2006, at a depth of 2,315 ft. Production started about 2 years later, through two 6.2-mile pipe-in-pipe flowlines with insulated steel catenary risers and an electrohydraulic control umbilical. The pipe-in-pipe design was chosen to minimize heat loss to the sea. The single well had the capability to flow through either or both flowlines, which were looped so that the operator could run a pig through them (Fig. 1). In addition, the well was equipped with umbilical tubes which allowed for methanol injection at the wellhead, downhole injection of a paraffin inhibitor, and a low dosage hydrate inhibitor, Goodman said.
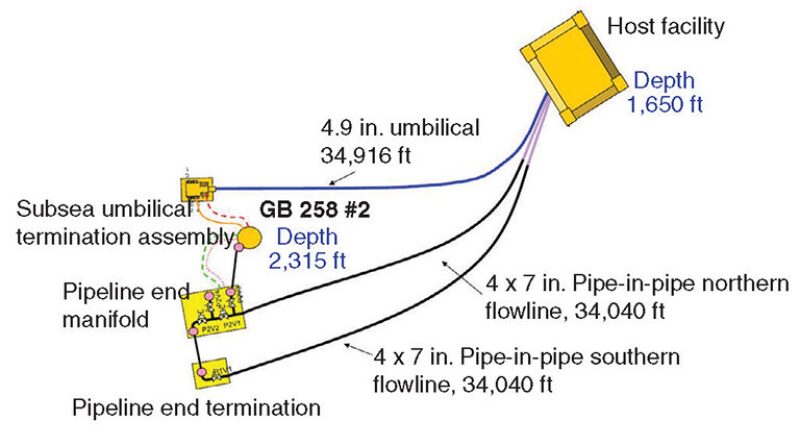
About 18 months after production started, the operator split the flow through the northern and southern flowlines to decrease the pressure drop and increase production. Initially, both lines operated at similar temperatures and pressures, but about 1 year later, the operator noticed some differences between the two. The temperature of the northern flowline was about 60°F, below the wax appearance temperature (WAT) of the crude, which was 106°F. Meanwhile, bottomhole pressure was building, and the total production from the two flowlines had declined by 1,000 B/D . The operator concluded that the northern flowline was partially plugged with paraffin, Goodman said.
To mitigate the paraffin deposits in the northern flowline and riser, the operator shut in production and used circulation pumps and a hot oil tank to circulate from 370 to 390 B/D of oil heated to about 150°F through the looped lines. Unfortunately, oil circulation flow rates were low due to equipment concerns and because the plug was so far from the surface facility. The hot oil treatment did not unblock the line. The operator halted the paraffin mitigation efforts because it was simultaneously drilling in nearby areas and had limited space available for equipment and personnel on the host platform.
A crew later returned to inject xylene into the northern flowline, and to remove it from the southern flowline. Production from the field was again shut in for about 2 weeks while this technique was applied. The goal was to dissolve the paraffin until a xylene injection rate of about 1.5 bbl/min was achieved, followed by pumping of heated water (180°F) through the loop system for additional cleaning, and flushing of the line with a pig.
The partially plugged line did not respond as expected to the xylene injection. Pressure built up in the northern line as injection started, indicating the line was completely blocked. The operator then tried to inject xylene into the southern flowline and to take returns from the northern flowline. But pressure quickly built up in the southern line, indicating the block in the northern line was not moving. Xylene injection failed to clear the line. The northern line remained blocked, but the operator learned the obstruction was about 15,000 ft from the wellhead, Goodman said.
Production from the well was routed through the southern flowline at a reduced rate. Because of the block in the northern line, the operator monitored the temperature and pressure of the fluid arriving from the southern line (installing a third line was not economical).
Within a few months, the fluid temperatures fell below the WAT, pressure was building at the wellhead, and flow from the reservoir was reduced—indicating that the southern line was beginning to plug.
The operator flowed the production from an upper zone (Zone A), which had been shut in. The production rate from Zone A was 4,200 B/D compared with the lower Zone B production rate of 1,200 B/D of total liquids.
Zone A was unproduced and, therefore, had a higher reservoir pressure, which resulted in an increased flowing temperature downstream of the choke, in excess of 180°F (Fig. 2). The temperature of the flow at the surface increased from 80°F to 133°F, exceeding the WAT (106°F).
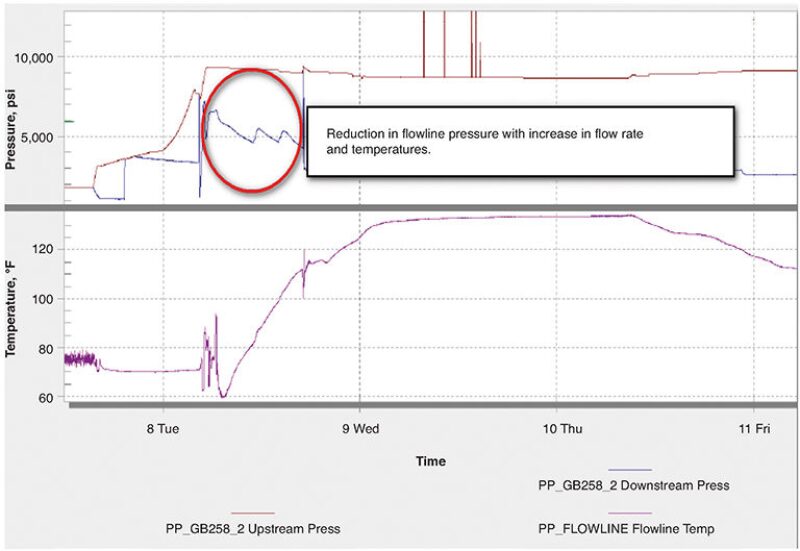
SPE Technical Section Addresses Flow Assurance
In response to the growing importance of flow assurance, SPE launched the the Flow Assurance Technical Section (connect.spe.org/fts/) in January. Chaired by Jeff Creek, Chevron ETC, the group is concerned with topics related to providing flow assurance between the reservoir and the processing plant.
Section officers include Phaneendra Kondapi, FMC Technologies; Steve Cochran, Chevron ETC; Hari Subramanian, Chevron ETC; John Nighswanter, Schlumberger; Dendy Sloan, Colorado School of Mines; Greg Hatton, Shell; Cem Sarica, University of Tulsa; and Scott Bufton, Intecsea.
Sarica, F.H. “Mick” Merelli/Cimarex Energy professor of petroleum engineering at the University of Tulsa, said that flow assurance is a broad field—a bridge between the reservoir and downstream business, particularly for offshore fields.
He noted that in general, the industry is doing a good job of developing deepwater resources and bringing hydrocarbons to the market. “It will all come from advances in technologies. Better technologies will open up new opportunities for us,” he said.
In developing the technical section, the group identified the need to facilitate interaction between industrial and academic practitioners. “We wanted to bring every stakeholder to the table and work together to enhance our understanding,” Sarica said.
The technical section provides a platform for sharing experiences of common problems to develop approaches to mitigate the problems. “We want to bring our heads together to see what we can do, what works, what doesn’t, and decide what technologies and best practices to pursue,” he said.
The group stressed that increased production and improved cost structures are the goals of effective flow assurance practices. For example, direct electrical heating of flowlines on the seafloor to prevent hydrate blockages and wax deposition is a technology that appears to be up and coming.
Heuristics related to solids deposition are being shared in efforts to develop best practices. For example, to determine where hydrates will be deposited, it is important to identify where free water occurs in a flowline and to minimize its volume early after the well. Development using subsea facilities offers a potential solution by decreasing the distance from the well to the processing facility.
The understanding of multiphase flow is considered to be prerequisite science for flow assurance problems because of the influences of local pressure, temperature, and fluid distributions. Sarica said multiphase flow prediction tools are generally developed using detailed data obtained from small-scale laboratory experimental facilities operated under low-pressure conditions (slightly above atmospheric pressures) and with limited high-pressure data. This can result in significant errors in prediction of multiphase flow. Therefore, the integration of higher pressures and larger pipe sizes into multiphase flow models is identified as an area for further improvement in flow assurance.
For Further Reading
SPE 166196 A Tale of Two Flowlines—Paraffin Plugging and Remediation by N.T. Goodman, Anadarko Petroleum Corporation and N. Joshi, Moulinex Business Services.
OTC 24396 Prevention, Management, and Remediation Approaches for Gas Hydrates in the Flow Assurance of Oil/Gas Flowlines by A.K. Sum, Colorado School of Mines.
SPE 163076 Experimental Study of Wax-Deposition Characteristics of a Waxy Crudy Oil under Single-Phase Turbulent-Flow Conditions by P. Dwivedi, C. Sarica, and W. Shang, University of Tulsa.
SPE 166335 Offshore Field Application of a Low Corrosive Fluid Designed for De-Scaling of Well with ESP Completion by H.B. Bakar, I.C. XianLung, M.Z. B. M. Nadzri et. al. Setegap Ventures Petroleum.