A new geothermal concept that blends oil and gas technology with emerging material science is set to undergo its first field tests later this year.
Called thermal reach enhancement (TRE) by its developer XGS Energy, the closed-loop approach seeks to generate cost-competitive geothermal power from standalone vertical or deviated wellbores.
The familiar part of the process involves flowing water—or another working fluid—down the wellbore before circulating it back up at a much higher temperature through insulated tubing to a standard turbine on the surface.
What distinguishes XGS from others in the revived geothermal sector centers on a proprietary material that it plans on pumping inside the hot rocks.
The Palo Alto, California-based company claims its TRE material is 50 times more thermally conductive than rock, which it anticipates will allow wellbores to absorb 30 to 50% more heat compared to standard geothermal wells.
“You can think of the material as a heat exchanger that brings the heat faster and more efficiently to the casing and then ultimately from the casing to the working fluid,” explained Ghazal Izadi, chief operating officer of XGS.
While details about the makeup of the conductive material are withheld to protect intellectual property, XGS plans to pump it downhole in a liquid slurry form at low rates. Flowing out from multiple perforations, XGS said the material will move anywhere from less than 1 m to as far as 10 m into the open fractures around the near-wellbore area before transitioning into its final solid state.
The perforations should seal off as a result of the solidification process and the expectation is that the fractures will close post-injection and compact tightly around the material—a step lab tests suggest is vital for maximizing its thermal conductivity.
In January, XGS announced that the idea attracted $9.7 million in an equity investment round led by US electricity provider Constellation Energy, bringing the company’s total raised since last year to more than $28 million.
The new funding will enable XGS to reach its next milestone which involves drilling a shallow well in Texas by the end of this quarter. Though the demonstration well will not be used to flow hot water, it will offer a dress rehearsal of sorts where the operational capabilities, including the crucial task of injecting the special material into the subsurface, will be tested.
The real final exam will come later this year when the company attempts to use its technology to reactivate an abandoned geothermal well.
By 2026, XGS hopes to be drilling commercial geothermal wells with outputs ranging from 3 to 10 megawatts thermal (MWt). Historically, geothermal plants convert about 10 to 20% of thermal energy into electricity, i.e., 10 MWt may translate to no more than 2 MW of electrical power. Presentations from XGS suggest using multiple wells to enhance electricity supply to the grid.
The company also estimates that the cost of electricity from its wells will compete with other geothermal and solar power sources. In scenarios using existing wellbores, the levelized cost of electricity is projected to not exceed $35/MWh, while new drilling projects may drive costs up to around $50/MWh.
More than a year of laboratory research and subsurface modeling work underpin these economic projections. XGS said it has tested the TRE slurry in various conditions using granitic rock samples, demonstrating its ability to withstand temperatures above 400°C (above 750°F).
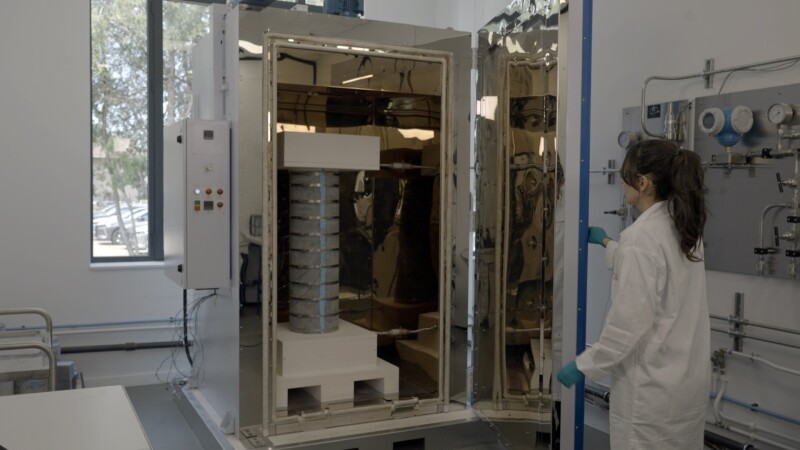
Izadi, a reservoir engineer and former global lead of geothermal for Baker Hughes International, said that the TRE technology holds several advantages over traditional geothermal along with the recently developed enhanced geothermal systems (EGS) that rely on hydraulic fracturing to connect pairs of injector and producer wells.
In contrast to these methods, XGS believes its closed-loop process offers greater geographic applicability as its wells should generate sufficient heat regardless of porosity or permeability since there is no reservoir fluid to flow. This feature also negates concerns over induced seismicity which most often occurs when faults in basement rocks are pressurized to a point of activation as the result of large-volume fluid injections.
When comparing its concept against EGS specifically, XGS asserts that it is looking at both a lower capex and a lower carbon footprint because it will not need to drill more expensive horizontal wells, source large volumes of injection water, nor use large hydraulic fracturing spreads to complete its wells.
Izadi compared the process of injecting the TRE material to the squeeze jobs used across the oil and gas industry to cement wells in place, which means a single pumping unit should be sufficient.
“We are going to squeeze this material very locally around the casing and leverage the microfractures in the rock or the preexisting natural fractures,” she explained.
When the time eventually comes to drill a new well, XGS is counting on the simplicity of basement rock to help manage costs.
While they are hot and often miles deep, which tends to drive costs up, the granitic and basaltic layers that XGS is most likely to target are also thick and homogenous. This may help contain drilling expenses by enabling the firm to forgo the use of technologies such as geosteering or other advanced well placement systems.
“We don’t need precision drilling—we just need to go deeper and deeper until we reach a certain temperature and then we can start to extract the heat,” said Izadi.