Nonaqueous drilling fluids, such as synthetic-based and oil-based mud (SBM and OBM, respectively), are used frequently to drill one or more sections of a well to reduce drilling problems such as shale sloughing, wellbore stability, and stuck pipe. However, solubility of formation gas in such fluids makes early gas detection, and the well-control process, very challenging. This is of particular concern in deep offshore wells. This paper presents a novel and comprehensive hydraulic model to simulate a gas kick in nonaqueous drilling fluids.
Introduction
Early kick detection becomes cumbersome when a nonaqueous drilling fluid is used.
×
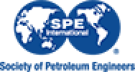
Continue Reading with SPE Membership
SPE Members: Please sign in at the top of the page for access to this member-exclusive content. If you are not a member and you find JPT content valuable, we encourage you to become a part of the SPE member community to gain full access.