As the shale sector looks for ways to improve well results, momentum is building to take a much closer look at how and where hydraulic fractures are created while pressure pumping fluids into tight, complex reservoirs.
The effort is being driven by unresolved questions over optimal well spacing and fracturing techniques. They are two closely related issues that dovetail into sector-wide production shortfalls associated to frac hits, a common well-to-well effect that experts in the technical community have recently named fracture-driven interactions.
One of the biggest challenges in overcoming these issues is to learn how to control the size of hydraulic fractures (the general emphasis is on reducing their lateral and vertical extensions) with a far finer degree of accuracy and finesse than is realistic today. There is an expanding array of diagnostic studies and new technologies working to this end. Several of the latest examples were highlighted at the recent Unconventional Resources Technology Conference (URTeC) in Denver.
Operators both large and small used the conference as an opportunity to express support for the broader use of tools considered to be classic components of petroleum and reservoir engineering: wellhead and bottomhole pressure gauges. These two technological cousins are nothing new to the oil field, but have only recently become viewed as essential among those seeking affordable answers about how their fractures behave during the treatment.
“The industry badly needs a low-cost, stage-by-stage method that we can use for assessing the reservoir quality, the completion design, and fracture complexities,” said Michael Sullivan, a reservoir diagnostics advisor with Chevron, during a technical session at URTeC. “Unfortunately, the high-cost and operational complexity is a barrier to most other stage-level assessments. What we need is something we can afford to do.”
Sullivan was presenting a paper (URTeC 970) that describes how Chevron’s Canadian asset team in the Duvernay Shale recently began using “free” wellhead pressure data to estimate each fracturing stage’s performance. His hope is that others follow the workflows as Chevron looks at more than half-a-dozen ways to use the data (including perforation cluster efficiency analysis and frac hit identification) to refine its completions approach.
Sullivan highlighted that the new learnings are thanks to pressure gauges it uses per standard procedure, meaning they are on wellheads whether the data is analyzed or not. To drive down costs further, Sullivan advised other operators to buy their own gauges vs. renting them from service companies. “What I’ve been emphasizing around our company is that this is a measurement we can afford to make—so let’s make sure we’re getting the most out of it,” he added.
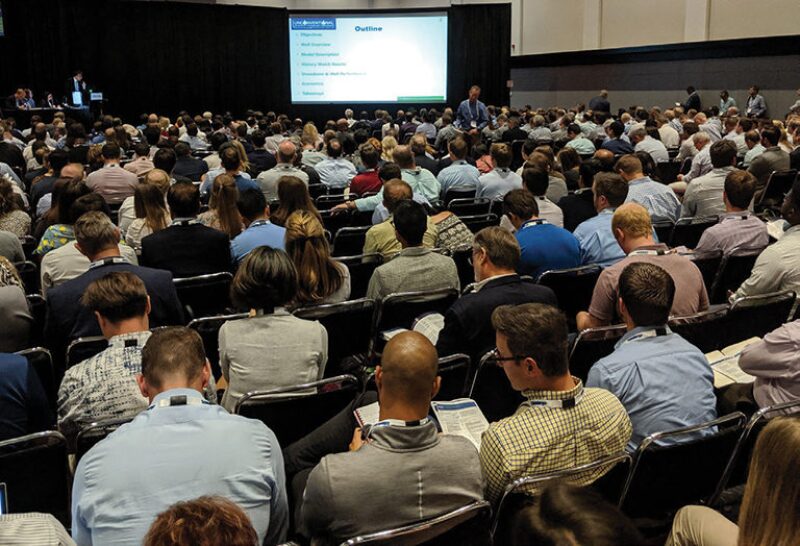
Thousands of petrotechnicals gathered in Denver for the annual Unconventional Resources Technology Conference (URTeC) where several new papers on advanced completion designs using new diagnostics were presented. Source: URTeC.
New Data Leads to New Directions
In the Permian Basin in Texas, Discovery Natural Resources is also paying close attention to well pressures measured during stimulations and documenting well-to-well interactions. The small, private oil company moved into a pure horizontal drilling program in the Wolfcamp Shale only 2 years ago and, not long after, it decided to start using pressure data from surface and bottomhole gauges to constrain fracture models and reservoir simulations.
The investment has delivered “some of the best datasets we have in the company,” according to Bryan McDowell, a technical advisor of asset development at Discovery. In two new papers (URTeC 125, URTeC 272), the operator describes how reservoir diagnostics and subsequent modeling have increased its understanding of fracture interactions and led to new completion designs based on landing zones for its stacked development.
There is one note of caution for companies just beginning to catalogue every recordable fracture interaction through pressure data: be prepared to take on the data management chores. In just one of Discovery’s recent fracturing operations, the pressure plots from the parent and child wells amounted to 56 million rows of data. “We’re a small company,” said McDowell, “so to us, it’s a huge amount of data.”
Neither Chevron’s Duvernay team nor Discovery have plans to use their new data to test completion designs that are tailored on a stage-by-stage basis, often called the “engineered” completion. However, several companies at URTeC revealed that they are in the hunt.
Despite various approaches, a universal objective of the engineered completion is to take into account the role of nature and prior reservoir production and then customize individual stage treatments to avoid extensive fracture overlap between wells.
Devon Energy is among those exploring this concept. In fact, the company is supporting research that aims to take things one step further by adding the “on-the-fly” element to the engineered completion. A new technical paper (URTeC 449) outlines its work with the University of Texas at Austin (UT) on near real-time fracture geometry estimation—a key steppingstone to making completion design changes during the treatment.
“To make real-time changes, we have to be able to make a real-time recommendation—and to make a recommendation, you have to understand what’s happening in the subsurface second by second,” explained Brendan Elliott. He is a senior staff completions engineer at Devon who is completing a PhD in real-time completion strategies.
The research team he works on at the university is seeking to prove out a new analytical model and a real-time inversion process that makes it possible to rapidly figure out where fractures are moving through the rock. Details of this model were shared earlier in the year by its principal developer, Ripudaman Manchanda, a research associate at UT, at an American Rock Mechanics Association symposium (ARMA 19-2071). The software will eventually be available for any company to license from UT.
The aim of the emerging toolset is to process enough data from a fracturing stage to inform changes on the subsequent stage. This could be done to optimize hydraulic fracture growth from a treatment well, or to prevent a fracture from breaking its way into the low-pressure zone of a produced well.
This would constitute the highest degree of fracture control ever achieved—but it is not a reality.
In presenting the conference paper, Elliott acknowledged such a vision of real-time completions is at least couple of years away. The evolutionary steps begin with making well-to-well changes, then stage-to-stage changes, and finally there may be enough confidence to test intra-stage changes.
All of this will require more field work to decipher which toggles are the biggest influencers of fracture growth and complexity—which could be treatment rates, how fast the rate is ramped up, total sand and fluid volumes, fluid rheology, cluster spacing, and the number of perforations in a cluster. Experts believe there are at least a dozen other factors, not least of which are the landing zone and near-field geomechanics.
Operators including Devon have already figured out how to manage at least one of these factors by adopting limited entry designs, also termed extreme-limited entry, which use a small number of perforations in each fracturing stage. Fewer perforations make it easier to assess cluster efficiency, while at the same time, those making the switch have also found that too many perforations can lead to fractures that are longer than desired, increasing the risk of unwanted frac hits.
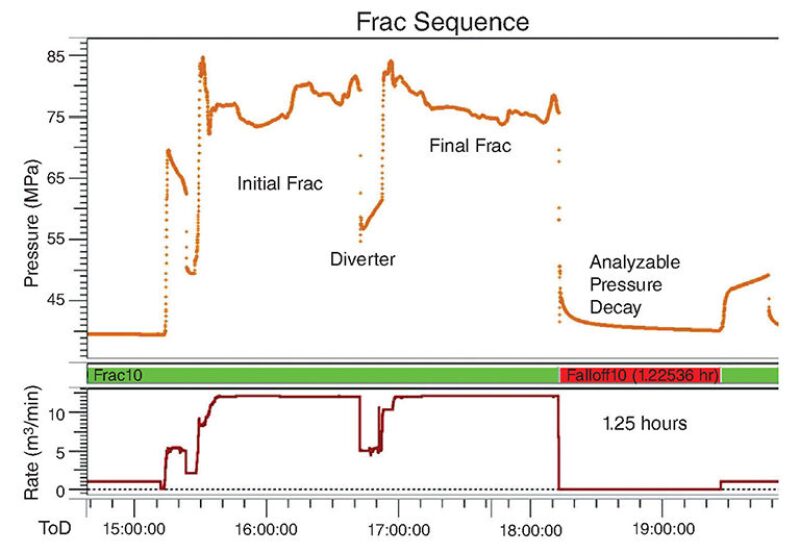
A Preference for Pressure
As operators look for reservoir diagnostics, they are finding that the most scalable tools are downhole and surface pressure gauges. Petrotechnicals working at Chevron’s Duvernay Shale asset in Alberta are among those following this emerging trend.
Measured during zipper fracturing, the supermajor believes that analyzing post-fracture pressure falloff data could unlock a slew of valuable insights.
“The basic premise is that when a fracture is connected to greater permeability—system permeability—the pressure will decay at a higher rate,” explained Sullivan while presenting the company’s paper at URTeC. “So when we can keep everything else constant, and change only one variable at a time, such as the fracturing fluid, geologic landing zone, completion type, etc., we can use this pressure fall-off rate as a comparison metric for optimization.”
As of the conference, Chevron had recorded the data from about 1,800 stages in 58 wells, on 15 pads—but only analyzed about half. The company is now working on automating the signal analysis to speed the process. While Sullivan stressed that Chevron’s understanding of the fall-off data is still “evolving,” and it has made no changes to its completions yet based on the “signal,” it has identified several ways it could lead to new plans and designs.
One finding Chevron shared was that the pressure data can reveal cluster efficiency—something shale producers have struggled to determine over the years. After validating with downhole fiber optics, the key takeaway is that when only a small number of perforations are taking fluid into the reservoir, a smaller pressure decay is observed after the stage is finished. Sullivan advised using the signal as a proxy to cluster efficiency “which then you could use for improving by changing your perf design, for example.”
In another experiment, Chevron analyzed the pressure data from five stages in a well completed using single entry point sleeves to eliminate the uncertainty of cluster efficiency. The stages landed in two types of rock: one was more brittle as defined by Young’s modulus, and the other was less brittle. Each saw significantly different leak-off rates, with the more brittle rock seeing the greatest pressure decay.
Chevron was able to conclude that the brittle rock stages saw more complex fracturing due to the higher rate of pressure leak-off. This was a major revelation for the asset team since the landing zone on these stages were all within 2–3 m of each other’s vertical depth. Previously, such small variances had not been seen as important factors for completions design.
“Actually, it’s a big deal,” emphasized Sullivan. “The brittleness of the rock can control the fracture initiation complexity, so what is within a meter of the wellbore may matter a lot.”
Chevron’s potential uses of pressure decay signal:
- Fracture modeling: Chevron is integrating the decay signal into geomechanical simulations to better understand its transient behavior.
- Frac hit verification: When zipper fracturing on a multiwell pad with a “plug and perf” completion, it may not be possible to know when a frac hit has occurred if the interval of the well being hit has already been isolated with a bridge plug. However, a frac hit is recognizable in the well being stimulated by observing an anomalously high rate of post-frac pressure decay.
- Geologic controls: Expectations are that the pressure decay signal correlates to rock brittleness and the presence of natural fractures. Chevron also wants to map the results spatially to understand regional geology.
- Proxy for production logging: If the rate of change in the signal indicates connected reservoir permeability, a stage-by-stage comparison may also indicate where the production is to flow from—thus, potentially serving as a reasonable alternative to using expensive production logging surveys.
- Image log correlation: The decay rate might correlate with the density of natural fractures, however the analysis has not yet been run on any wells that used image log data along the lateral section.
Why Real-Time Completions Won’t Happen
Customizing stages—whether planned or made during a treatment—is considered by many operators as imposing too much work on technical staff, which in many cases is doing more work with fewer resources. Most shale producers have shrunk considerably since the heady days of $100/bbl oil, and many continue to downsize as the sector faces ongoing financial hardship.
And between the limited number of companies implementing or testing engineered completions, recent industry literature and conference-circuit dialogue on the topic suggests that more producers are comfortable with planned changes than those that think they can make them during the treatment. Time will tell if the current research results in more converts.
“Currently, industry does not see a clear benefit to making changes to the pumping schedule on the fly, because we don’t really know how to do it,” explained Mukul Sharma, a petroleum engineering professor at UT and proponent of on-the-fly changes. He is also involved in the research project with Devon.
Despite the current state of readiness, Sharma argues that with infill drilling representing an ever-growing share of the North American shale well inventory, real-time or near real-time fracture control will be an increasingly important tool for avoiding negative fracture interaction. “As the technology develops and we improve our ability to interpret inexpensive observations in adjacent monitor wells, I believe we will see the benefits of doing things this way.”
The opposing viewpoint is that on-the-fly changes pose unnecessary challenges and that it is simply incompatible with the industry’s adopted “factory mode” approach.
One of those hurdles involves change management. Shale producers are famously rigid in how little flexibility is given to the fracturing consultants and company men tasked with carrying out plans made weeks or months prior. This bleeds into accounting issues that would arise since final investment decisions on new wells are also made along the same timelines. Changing well designs on the spot makes it difficult to project costs.
David Cannon, the senior vice president of geoscience and technology for Diamondback Energy, was one of several oil and gas executives at URTeC who raised other concerns. His centered around how on-the-fly completions would expose delicate supply chains that operators have spent years establishing, along with the “safe and efficient” work routines enjoyed by fracturing crews and those that pay their day rates.
The gamble is that disrupting this fine-tuned orchestra of capital and labor risks negating any positive economic benefits achieved in the reservoir.
“I think it’s an admirable goal,” Cannon said of on-the-fly completions. “However, at the same time, you always have to think about the efficiency of the operations at the surface. When you can become repetitive, you can become very efficient.”
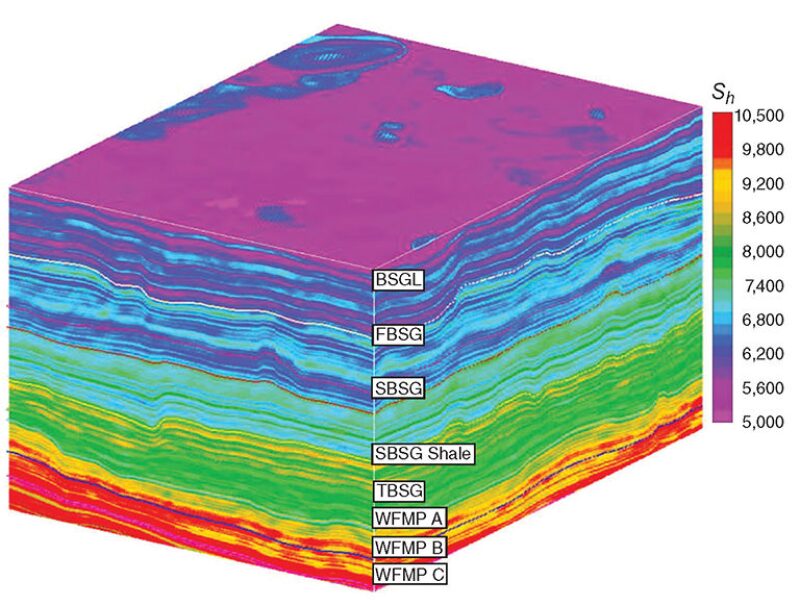
Ready or Not, Here They Come
While engineered completions remain a larger point of debate than they do an industry practice, some operators are at least testing the waters.
Callon Petroleum, a mid-sized Permian operator, is placing its bets around a mechanical Earth model and seismic inversion to predict how fractures will propagate in different layers of rock. The company’s paper (URTeC 208) notes its motivation to experiment with engineered completions stemmed from concerns about the sustainability of completions using “a repeated sequence implementing identical geometric stage placement and pumping schedules.”
By characterizing rock stress with logs, cores, and seismic data ahead of time, Callon has found that in high-stress zones, fracture growth is more likely to be contained, resulting in less well-to-well interference. In low-stress zones, engineers and petrophysicist are using a new “seismic-to-simulation” workflow to avoid fracture asymmetry and frac hits by using wider stage spacing and less aggressive stimulations. Callon says these learnings have enabled it to high-grade its acreage based on rock stress.
Equinor is also in the early stages of studying rock stress for engineered completions at its Eagle Ford Shale asset, but it is taking a different route than Callon. Instead of using seismic interpretations, the company presented a case study it says validates the use of surface drilling data—i.e., mechanical specific energy (MSE)—and a new modeling software to infer pore pressure and stress states (URTeC 511).
Low pressures and stresses are correlated to areas of reservoir depletion, which as mentioned earlier, should affect stage placement and treatment. Equinor points out that since the MSE data is already recorded during drilling, its acquisition comes at no extra cost.
“A Direct Known Point in Space”
As more operators realize that pressure data is reflective of fracture geometry, some want to get as close to the source of that pressure as possible—the fractures themselves. Devon is among those taking pains to do just that.
One of the company’s URTeC papers that it coauthored with researchers from UT describes efforts to create a “new, fast analytical method to estimate fracture geometry, and the resulting stresses around a propagating fracture” by identifying poroelastic responses that are the result of stress shadowing. Unlike other recently established pressure-based diagnostics, this innovation forgoes using bridge plugs to isolate and monitor a particular fracture stage in an offset well.
Instead, the unique approach calls for bottomhole pressure gauges to be installed at various locations along an offset well’s lateral section. These gauges are “externally ported” to the rock formation to measure pressure in the reservoir and prevent pressure communication with the inside of the casing.
The closer each gauge is to a propagating fracture correlates to higher-observed pressures. The extra effort of using downhole gauges this way is done to enhance the certainty of the reservoir’s pressure response data by attaching it to “a direct known point in space.”
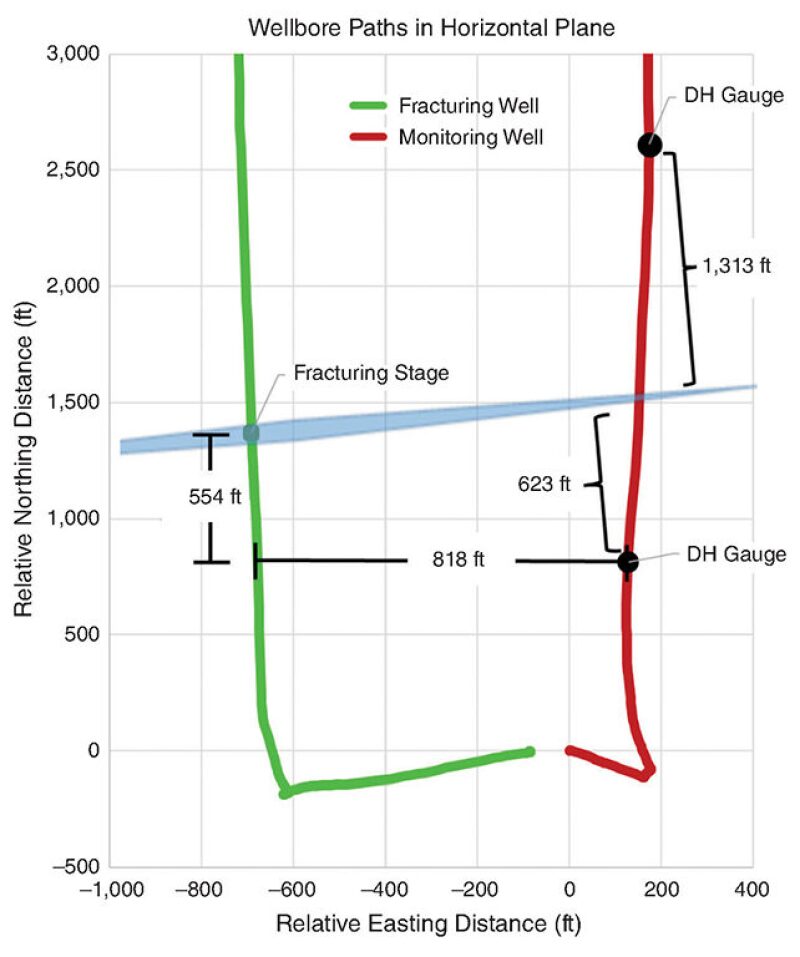
The horizontal wellbore trajectories and distances between treatment stage and monitoring points used in a Devon Energy pilot of a new pressure diagnostic method. Due to the azimuth of this fracture and wellbore geometry, a fracture was observed passing the heel downhole gauge before a response was seen in the mid-lateral gauge. Source: URTeC 449.
Analysis of the data is updated continuously as a stage is pumped, which allows engineers to use it as a near real-time method for visualizing the pressure growing inside the formation as the fractures move through the reservoir.
Devon has validated the new model using more complex 3D- and geomechanical-coupled numerical simulators, which are useful, but not in the context of real-time decision making since they take hours or days to run. The new analytical model only takes seconds.
“I think this is incredibly powerful,” said Elliott. “We really haven’t had this before, and the ability of simple models to project this is quite impressive.” He added that as the picture of fracture behavior and reservoir pressure changes becomes sharper, designed experiments can be run to test different parameters of the overall completion strategy.
The central job of the new model is to help figure out the three key unknowns of fracture geometry: net pressure, fracture height, and fracture half-length. The challenge is that for any given pressure response, these three parameters can be combined in a nearly infinite number of ways to match the response. This means a fracture could be close to the point of measurement, or it could be farther away, and of different sizes, while generating the same observed pressure response.
To get closer to the true answer, the UT research team has turned to an inversion algorithm that Elliott said enables the software to “calculate the full solution space” which is displayed as a curved surface. Each downhole gauge provides one of these curves. When three gauges are used, the most likely solution for net pressure and fracture geometry is triangulated.
In the first pilot, Devon only used two gauges. One gauge was placed in the heel zone and one in the middle of an offset monitoring wellbore. At 8 minutes into the fracturing of an offset stage, the heel-gauge recorded a pressure rise while the mid-lateral gauge did not see a response until 24 minutes into pumping. Knowing where these points were in the well, and how far apart those responses were in time was all that was needed to reveal the azimuth and behavior of the fractures.
The next set of goals for the Devon and UT team will be to run more pilots, validate the model against data sets from other industry partners, integrate the data with other diagnostic technologies, and move the process into real-time mode.
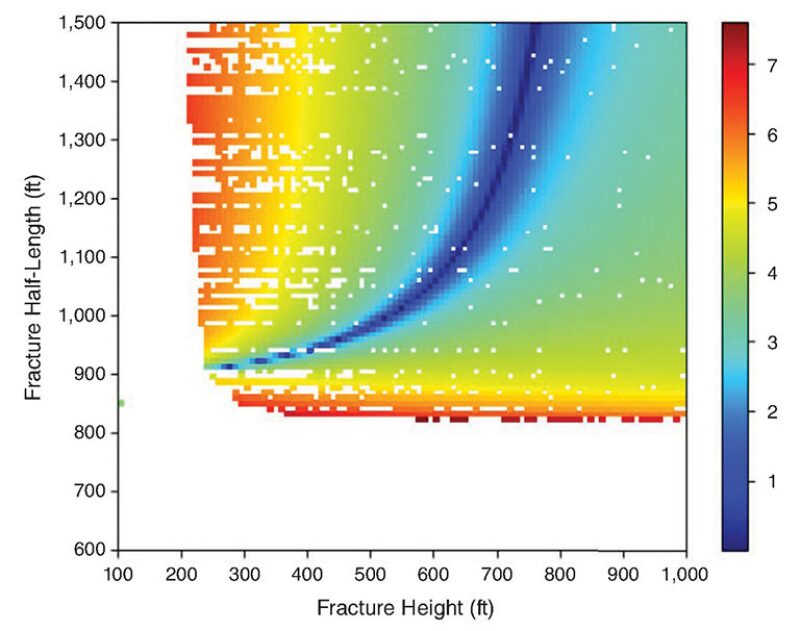
Water Hits, Arrival Times, and Pinballs
The work of detailed reservoir diagnostics is not the sole domain of large companies. There are ample case studies that show how small producers have increased their scrutiny of reservoir pressures and adopted integrated modeling workflows to engineer better well designs.
One of the latest comes from Discovery, which has only about 200 employees. In just 4 months, a single person in the company was able to develop a frac hit workflow to diagnose the interactions it was observing by correlating production effects. The reservoir simulation workflow, which incorporates some of that data, took a year to build with four staffers.
Among the most critical learnings of this combined reservoir diagnostics program is that frac hits in Discovery’s location tend to have neutral effects on oil production, enabling the company to tolerate them with only slight delays in daily production.
This was realized using data from downhole and surface gauges that in some cases recorded pressure increases in shut-in offset wells by as much as 2,000 or 3,000 psi during the treatment of another well. These pressures were often sustained for days and weeks.
Despite the magnitudes, pressures often built up slowly, almost to the initial reservoir pressures recorded before the first offset wells were hydraulically fractured. When production data came in later, the engineering team saw something they never expected: gas-to-oil ratios (GOR) in the offset wells went down.
“With frac hits, what we see is a bump in water production and a drop in GOR—every single time without fail,” said McDowell at Discovery, explaining that the working theory is that the fracturing fluids are pushing the gas back into solution, providing a stronger drive for oil production once the well cleans up from a few days or weeks of solid water production. “It was pretty eye opening,” he added. “We knew things were happening, but we never knew the extent of the pressure.”
Others have seen similar responses in the Permian and elsewhere, with one theory being that a slow offset pressure buildup indicates a complex fracture network with many pathways for fracturing fluids to travel. Some experts believe that the tortuosity of these complex systems is such that the proppant gets held up somewhere in the near-field of the fracture network, while the injected water is able to flow much deeper into the formation and adjacent wellbores.
Especially in water wet geologies, which includes Discovery’s position in the Wolfcamp, this becomes a plausible explanation of why wells that take frac hits are spared from being sanded in, meaning no proppant is observed. Instead they produce a large volume of water for several days or weeks before returning to baseline oil production. Accordingly, the company refers to these events as “water hits.”
Discovery has inferred other interesting fracture behaviors from pressure data, including how fracture interactions seem to relay from one well to another like a “pinball,” described McDowell.
Related to this, and in a fashion similar to Devon’s (but relying on the traditional application of gauges and commercial software), Discovery has shared how it determines the average velocity of fracture growth by measuring how fast the pressures move from well to well. The team uses this data for enhanced frac hit detection and well interference testing since it can learn the tendencies of its rocks by using the velocities to calculate the “average arrival time” of each stage’s fractures.
The case study proves that knowing when a pumping stage begins, the distance between wells, and the moment when offset pressures rise, an operator of any size can see how fast fractures are propagating outward from the treatment well. As these patterns are seen over and over in the same field, the data becomes a hard-to-ignore diagnostic for well spacing.
One reason why all these nuances are so important to document is that Discovery needs them to make its investment in reservoir models and hydraulic fracturing simulators worthwhile. These tools are seen as critical to making informed changes to completion styles. However, many small and mid-sized operators have shied away from models and simulators due to their cost, complexity, and uncertainty.
But with low-cost pressure data, operators have at least one constraint to make their models and simulations more useful and believable. McDowell and others still know that getting the exact prescription for well spacing remains a difficult task. “But cutting down uncertainty is absolutely possible,” he said. “And if you can cut down uncertainty enough, then you’re eventually left with the answer, or at least a better answer than you started with.”
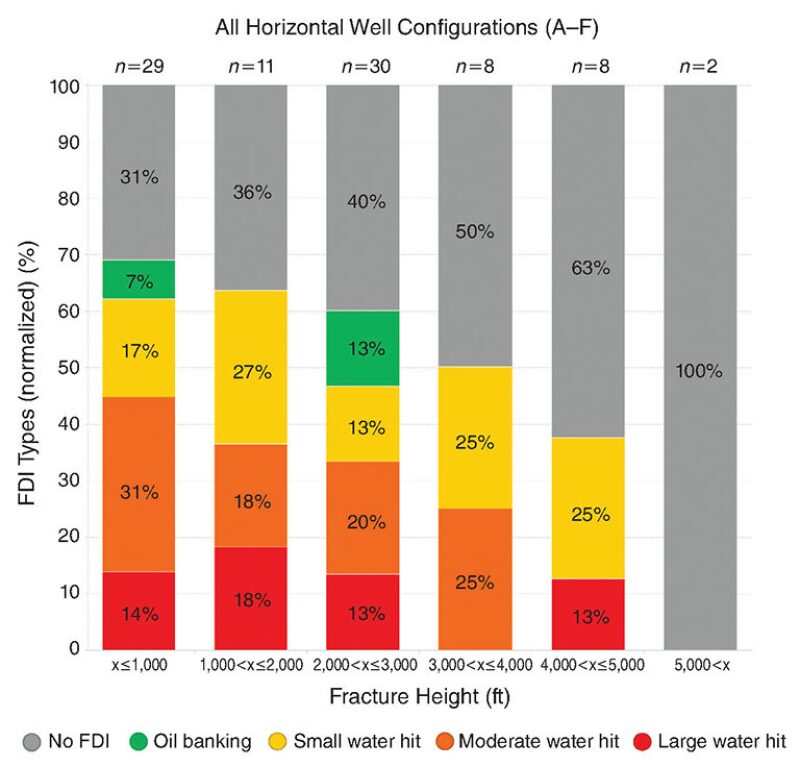
For Further Reading
URTeC 511 Estimation of 3D Distribution of Pore Pressure from Surface Drilling Data—Application to Optimal Drilling and Frac Hit Prevention in the Eagle Ford by James Kalinec, Equinor, Mohit Paryani, FracGeo, et al.
URTeC 208 Frac Hit Prevention and Engineered Treatment Design in the Permian Basin Using In-Situ Stress From 3D Seismic by Michael Shoemaker, James Hawkins, Callon Petroleum Company, et al.
URTeC 449 Interpreting Inter-Well Poroelastic Pressure Transient Data: An Analytical Approach Validated with Field Case Studies by Brendan Elliott, Devon Energy, Ripudaman Manchanda, The University of Texas at Austin, et al.
URTeC 970 Post-Fracture Decay: A Novel (and Free) Stage-Level Assessment Method by Michael Sullivan, Chevron, Behnam Zanganeh, University of Calgary, et al.
URTeC 272 Practical Reservoir Simulation for Small Development Teams—Customizing Stimulation Designs by Landing Zone in the Midland Basin by Tony Tran, Ryan Miller, Discovery Natural Resources, et al.
URTeC 125 Production Effects From Frac-Driven Interactions in the Southeastern Midland Basin, Regan County, Texas by Bryan McDowell, Alan Yoelin, Discovery Natural Resources, et al.