Proponents of carbon-free nuclear energy have envisioned mass-produced small modular reactors (SMRs) that generate less than 300 MW and can be transported from place to place by truck as a cheaper, safer option to traditional gigawatt-scale light water reactors (LWRs).
But some researchers contend that current SMR designs may create more and somewhat different waste disposal problems than their big cousins.
A recent study, published in Proceedings of the National Academy of Sciences (PNAS) in May by researchers at Stanford University and The University of British Columbia, assessed three SMR designs and concluded that issues related to the management and disposal of the nuclear waste they create surpassed that of a 1,100-MW pressurized water reactor by factors varying from 5 to 35.
“These findings stand in sharp contrast to the cost and waste-reduction benefits that advocates have claimed for advanced nuclear technologies,” study lead Lindsay Krall, a former MacArthur Postdoctoral Fellow at Stanford University’s Center for International Security and Cooperation, told Reuters.
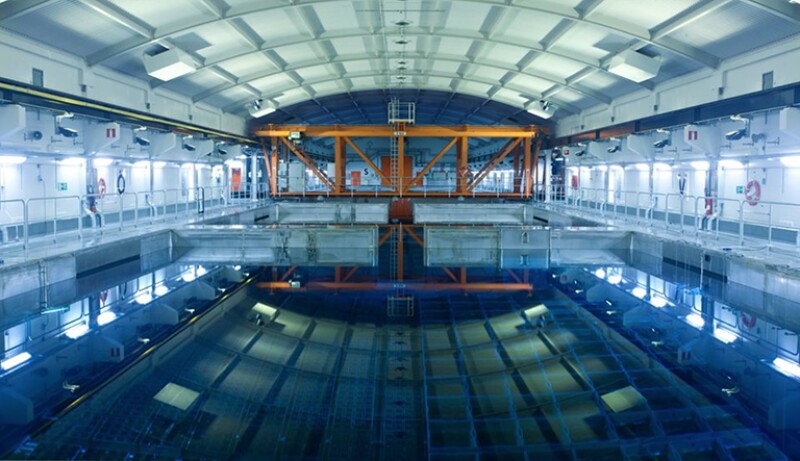
Krall is a scientist at Sweden’s SKB, the Swedish Nuclear Fuel and Waste Management Company, which manages and stores radioactive waste for the country’s nuclear power plants. Sweden gets 40% of its electricity from nuclear, 40% from hydropower, and 10% from wind, thus earning bragging rights as the EU country with the lowest level of carbon dioxide per kWh of produced electricity, according to data published in July by the European Environment Agency.
A 2018 MIT study concluded that virtually carbon-free nuclear energy is so vital to decarbonization that costs to achieve climate goals will be 50% higher if nuclear is removed from the energy mix.
The Stanford/British Columbia study assessed three SMRs, each with different cooling system designs: Fluor-backed NuScale Power’s water-cooled advanced reactor, the first SMR to receive key approvals from the US Nuclear Regulatory Commission; Terrestrial Energy’s molten-salt-cooled system; and a sodium-cooled SMR designed by Toshiba.
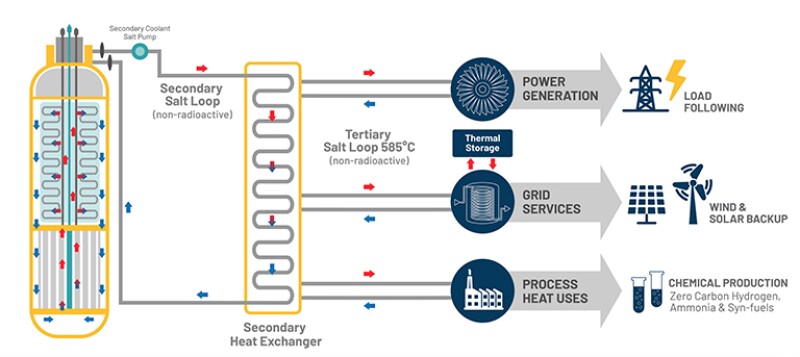
Researchers corroborated results of the three reactor assessments with theoretical calculations and a broader design survey. This led them to conclude that the dozens of SMRs designs now in various phases of R&D are more likely to leak neutrons, making these designs, as stated in the report, “ … inferior to LWRs with respect to the generation, management, and final disposal of key radionuclides in nuclear waste.”
There are currently no SMRs in operation and since their designs tend to be proprietary, the study’s authors admitted to having had difficulties in making their analysis.
One of the more advanced SMR projects, NuScale’s, developed with $400 million in US Department of Energy funding since 2014, won’t begin to generate power until 2029 when it expects to deploy the first of six 77-MW modules as part of the Carbon Free Power Project in partnership with Utah Associated Municipal Power Systems. The NuScale plant is to be built at the Idaho National Laboratory near Idaho Falls.
Another subject of the study, Terrestrial Energy, took PNAS to task within days of publication. Terrestrial’s Chief Technology Officer David LeBlanc addressed concerns over what he called “numerous and significant factual errors” made in the report, in a letter dated 3 June to the publication’s chief editor.
University researchers noted that “the low-, intermediate-, and high-level waste stream characterization presented [in their findings] reveals that SMRs will produce more voluminous and chemically/physically reactive waste than LWRs … because of “neutron reflectors and/or chemically reactive fuels and coolants” used in SMR designs.
Thus, not only is the volume of waste a problem, but more importantly the very different types of waste created in an SMR core which—because of its small size—is likely to leak neutrons that normally sustain the necessary chain reaction into surrounding structural materials such as steel or concrete.
SMR Waste Disposal: A Novel Engineering Challenge
Neutron leakage affects the properties of reactor waste, and spent fuel from an SMR has a relatively high concentration of fissile nuclides whose criticality must be identified during storage and disposal. For example, molten-salt- and sodium-cooled SMRs employ “highly corrosive and pyrophoric fuels and coolants that, following irradiation, will become highly radioactive,” the study pointed out.
Relatively high concentrations of isotopes plutonium-239 and uranium-235 in SMR spent nuclear fuel will render “recriticality” (a return to a point at which a nuclear reaction becomes self-sustaining). This, the researchers said, is “a significant risk for chemically unstable waste streams.”
Moreover, SMR waste streams aren’t suited to direct geologic disposal if they are prone to exothermic chemical reactions or become critical when brought into contact with water.
Among points raised in his rebuttal letter to PNAS, Terrestrial Energy’s LeBlanc noted that “no reactor uses or proposes using pyrophoric [able to ignite spontaneously on exposure to air] fuels” and “while sodium reactors indeed have a pyrophoric coolant, the coolant does not become highly radioactive.” Any wording from the study that implies “both molten-salt and sodium- cooled reactors have pyrophoric fuels and coolants … is simply false,” LeBlanc wrote.
Still, researchers contend that the radiotoxicity of plutonium in spent fuels discharged from the three SMRs studied would be at least 50% higher than the plutonium in conventional spent fuel per unit energy extracted 10,000 years after interment.
DOE Tees Up a New Round of Grants
All of this is hardly news to the DOE which has been funding R&D of various SMR designs over the past 10 years with an eye to bringing the first projects online before 2030.
Waste management is part of the R&D agenda and in March it took center stage when the DOE announced a new $48-million round of research grants to develop safe and sustainable management of SMR waste as well as other technologies to support the DOE’s Advanced Nuclear Reactor technology initiative to support high-impact technologies that aren’t yet ready for private investment.
Aptly called CURIE (after the Nobel prize-winning scientist Marie Curie who discovered radium), the DOE program offers grants to develop innovative separations technologies, online monitoring, and materials accountancy methods to support domestic US production of advanced reactor fuel feedstocks or important commercial radioisotopes and critical minerals.
CURIE aims to support research to design systems to power SMRs with recycled unused (or spent) nuclear fuel, separation technologies to mitigate the proliferation of nuclear material, and minimize the production of waste requiring permanent disposal, according to the DOE.
Simply put, the goal is to substantially reduce the heat load and radiotoxicity of waste requiring permanent disposal while simultaneously creating a sustainable fuel feedstock for advanced fast reactors by recycling spent fuel.
In March the DOE also dispersed $36 million to 11 research teams under a related government program, ONWARDS (Optimizing Nuclear Waste and Advance Reactor Disposal Systems). The largest of those grants, $8.5 million, went to the Washington state-based nuclear innovation company TerraPower to further research on an experimental method to recover uranium from spent nuclear fuel utilizing the volatility of chloride salts at high temperature.
The US is the world's largest producer of nuclear power, accounting for more than 30% of worldwide nuclear generation of electricity, according to the UK-based World Nuclear Association. The US claims 93 of the 440 nuclear reactors that produce electricity globally, with two more traditional megaplants due to become operational in 2023.
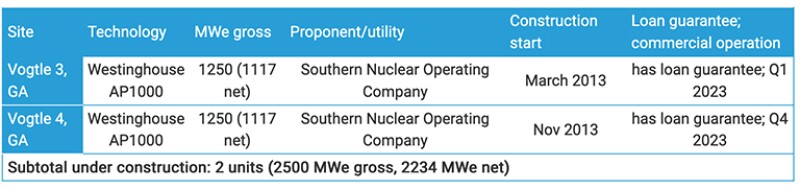
Already, traditional commercial nuclear power plants in the US have piled up more than 85,000 metric tons of spent nuclear fuel and related waste requiring isolation in deep-mined geologic repositories for thousands of years, and new waste continues to build at reactor sites at a rate of 2,000 metric tons per year, according to the US Government Accountability Office.
No country has yet to construct a final repository for spent nuclear fuel, though Finland, Sweden, and France are so far the most advanced in the process, according to SKB. The US shelved the Yucca Mountain site in Nevada as a solution years ago and is yet to come up with an alternative.
Finland, however, is on track to open the world’s first spent fuel repository in 2023 using storage and interment methods developed by SKB: copper canisters with spheroidal graphite cast iron inserts, each containing 2 tons of waste. Bentonite clay surrounds the canisters which are placed 500 m deep in Precambrian bedrock.
The volume of nuclear waste produced so far by Finland and Sweden (2,300 tons and 8,000 tons, respectively) pales, however, when compared to that of the US; hence the DOE’s emphasis on funding research into methods of recycling unused nuclear fuel to power SMRs rather than storing it all for tens of thousands of years.
Posiva, a joint venture between Finnish nuclear energy operator TVO and the country’s Fortum energy company, is building the repository and expects to bury its last spent fuel waste canister 100 years from now. Sweden plans to open its own final resting place for permanent burial in the early 2030s.
Meanwhile, SKB and Posiva leave open the possibility that new technologies may emerge over the next century enabling them to exhume some waste if recycling of spent fuel becomes a reality.
_______________________________
A Nuclear-Powered Moon—Less Controversy, More-Complex Tech
NASA said it has chosen three design concepts to develop nuclear fission power for its Artemis moon base, with each to receive $5 million in funding to the DOE’s Idaho National Laboratory.
The 12-month contract announced in June aims to further develop designs for 40-kW-class nuclear fission power plants capable of operating for a minimum of 10 years on the lunar surface. Three consortia were chosen:
- Lockheed Martin (Bethesda, Maryland, consortium leader), BWXT, and Creare
- Westinghouse (Cranberry, Pennsylvania, consortium leader) and Aerojet Rocketdyne
- IX (a Houston joint venture of Intuitive Machines and X-Energy, and consortium leader), Maxar, and Boeing
Nasa pointed out that the successful demonstration of such a system on the Moon would open the way for long-duration Moon and Mars missions. The project would also help NASA to mature nuclear propulsion systems for spacecraft for use in deep space exploration missions, including crewed missions.
The European Union is also pursuing development of nuclear propulsion for spacecraft and has named Belgium’s Tractebel to take forward research on Europe’s production of plutonium-238.
Currently only the US and Russia have produced plutonium-238 which is used in spacecraft propulsion. The European Space Agency sees its production in Europe as critical to meet its goals of establishing its own moon base.
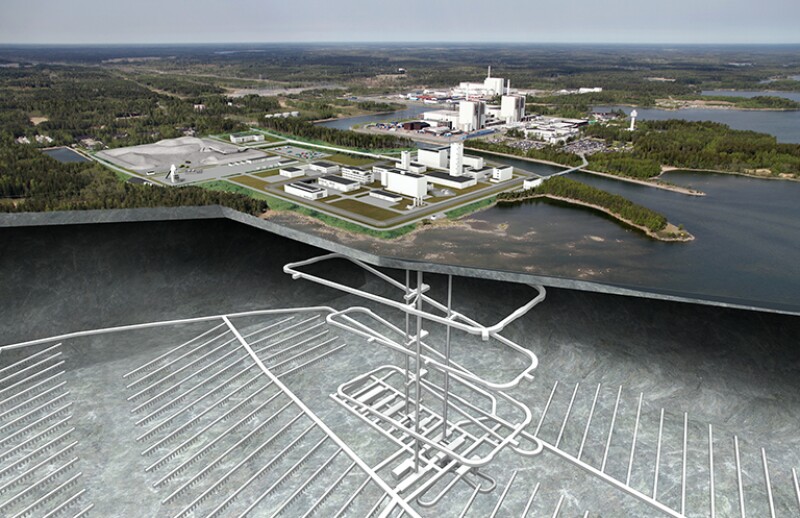