Development of the Eagle Ford shale typically consists of horizontal wells stimulated with multiple hydraulic-fracture stages. This paper presents a pragmatic integrated work flow used to optimize development and guide critical development decisions in the Black Hawk field. Geoscientists and reservoir and completion engineers worked collaboratively to identify optimal completion designs and well spacings for development focus areas. Multiple simplistic simulation models were history matched to existing production wells.
Introduction
In 2008, the operator drilled several successful wells in the Hawkville field of what would become the Eagle Ford shale play. Early results led to substantial land acquisition. The Eagle Ford, while continuous over wide sections, varies substantially in terms of fluid and rock properties. Fig. 1 shows a cross section for an arbitrary line through Black Hawk and Hawkville to the Maverick basin, showing the relative changes in thickness and Young’s modulus.
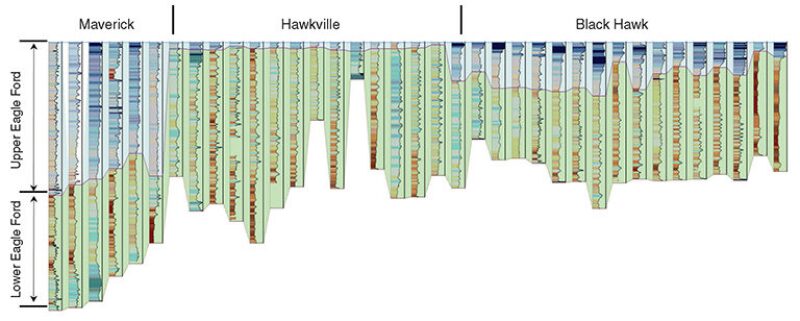
An understanding of the characterization of shale systems for simulation has evolved rapidly. Flow contributions from natural fractures, induced fractures, and matrix rock along with the nature of the hydrocarbon deposit itself should be considered. Perhaps even more important is regional variation. In the world of conventional assets, property estimation needs to be reliable only for a small geographical area, often within one sandstone structure of a few square miles at most. This can be compared with the scale of the play in Fig. 1. For conventional reservoirs, standardized laboratory methods and years of research and trial and error have educated our approaches to well-defined best practices. In shale plays, these have not yet been fully worked through and adopted by consensus, often leaving the owner of the asset as the arbiter of methodology.
Work Flow
The work flow proposed in this paper follows the advice of Carveth Read, the British philosopher, regarding uncertainty: “It is better to be vaguely right than exactly wrong.” Simple models with a multitude of geological scenarios are used to understand key drivers. From this starting point, the work can progress further through the complex and time-consuming approaches that can be performed only in a small subset of locations where data permit.
Fig. 2 shows the work-flow outline applied in the Eagle Ford fields to obtain timely estimates of reservoir response to spacing and completion design.
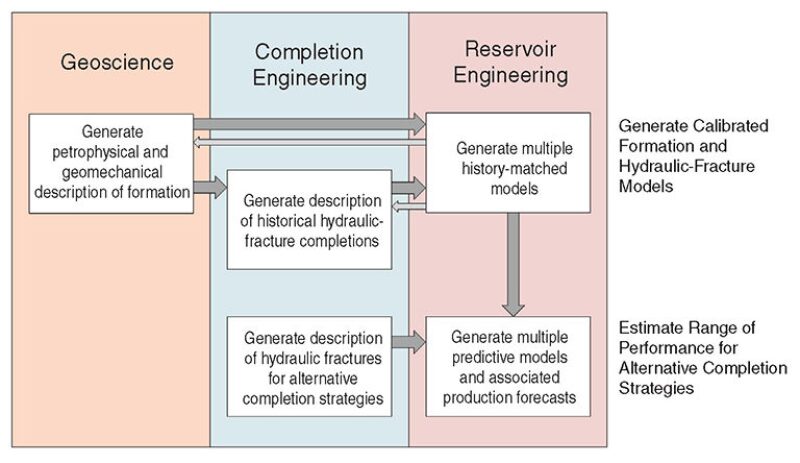
The fields were split into areas of geological and fluid similarity. For each area, a regional geocellular model was generated, incorporating local well-control, seismic, log, and core data. Within this region, an existing well with stable production history was selected for history matching. Average layer formation properties, areally upscaled to the estimated drainage region of the well, were extracted from the model for reference-case description of the hydraulic-fracture and dynamic-simulation models.
Expected fracture geometries and conductivities for the historical well completion were estimated in hydraulic-fracture models. These results were used to guide the definition of explicitly modeled hydraulic fractures in the reservoir-simulation models. Multiple history-matched models were generated with computer-assisted-history-matching techniques.
The unique contribution of this work is to illustrate the basis for making development decisions considering the inherent uncertainty in the forecasts. The reference description of the formation and hydraulic fractures was made on the basis of the extracted properties of the geocellular and hydraulic-fracture models, respectively. Stochastic realizations from ranges of key properties in formation and completion parameters were tested.
The resulting calibrated reservoir scenarios formed the basis of optimization studies for development drilling and down spacing. Completion-design parameters were evaluated in hydraulic-fracture models. The resulting fracture geometries were simulated, and the optimum completion design and well spacing were determined for each area.
For a geological overview of the Black Hawk field and associated petrophysical and multimineral work flows, please see the complete paper.
Fit-for-Purpose Geologic Modeling in Shale Plays
Development in unconventional reservoirs currently relies on horizontal wells designed to stay within a defined target zone and to follow along strata as closely as possible. The reality is that geologic layers are not perfectly continuous and formations change laterally in thickness, properties, and structure, especially near folds and small faults. As the use of horizontal wells in excess of 3,000 ft becomes customary, lateral changes in the formation become even more important. One of the principal factors in the successful development of unconventional reservoirs is the proper spatial position of horizontal wells within the vertical section of the target reservoir. A simple work flow to account for changing reservoir and geomechanical properties was developed to assist modeling and geosteering of the wells.
A high-resolution version of the reservoir model was constructed that could be upscaled for flow simulation. The design geometry of the upscaled model was based on the production well selected by the engineers for history matching in different areas in the field. The upscaled model incorporated all cells within the estimated drainage volume of the well, accounting for well orientation, length, and spatial position within the regional structural framework. The work showed that correct understanding of the position of the well relative to the gross interval of interest was often very important.
The framework of the geologic model was based on the preparation of robust regional structural maps tied to pilot and horizontal wells in the area and trended by depth-converted seismic horizons. Tops along the wells were quality controlled, with geologists geosteering the wells. Accurate placement of all available horizontal wells permitted distribution of reservoir properties using all available data. Total porosity was distributed by use of upscaled logs that varied in vertical resolution from 3 to 5 ft, depending on the geologic section. The distribution was a sequential Gaussian simulation using depositional axis as a trend in the variogram. This axis varied for each location to be studied. Water saturation and permeability were distributed in a similar fashion, with the difference being that total porosity was used as a secondary trend. Each geomechanical unit had a separate distribution.
Using seven geomechanical units, simulation-model grids were designed on the basis of each one of the wells to be evaluated and the reservoir properties in the high-resolution model. Both the high-resolution static model and the upscaled models honor available core data and petrophysical analysis of wireline logs, guaranteeing that the petrophysical analysis is honored through the entire process. This work flow is different from commonly used conceptual models in that the models of the former are fully tied to the regional framework of the area and the depth from which the well to be analyzed originates, without the time requirements of most complex geomodels.
Applying this fit-for-purpose approach, two critical objectives were accomplished: improved characterization of unconventional reservoirs as development-drilling plans were updated to reflect the optimal well spacing for each lease, and development of an active reservoir-modeling work flow that was integrated into the reservoir-simulation efforts as they were occurring simultaneously.
Completion Strategy
Most completions in the Eagle Ford have been either crosslinked (XL) gel or slickwater treatments. The operator elected to use XL-gel and XL-gel/channel-fracture stimulations on the basis of early experimentation in the Hawkville field. The majority of the wells to hold the acreage were completed with XL/gel-channel-fracture treatments. Table 1 shows the critical variables and ranges in selection across operators in the play.
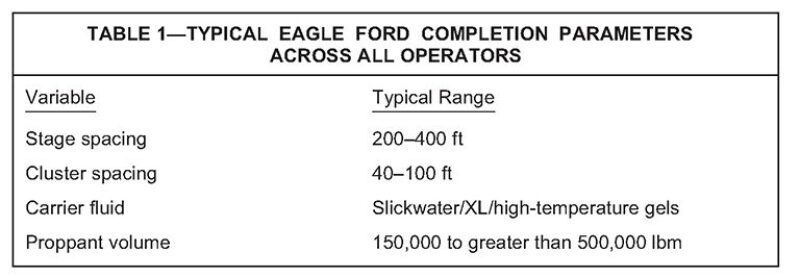
Well-spacing and completion optimization have a symbiotic relationship in development planning. Adjustments that potentially extend effective fracture half-length will have a direct link to the optimal wellbore spacing for the section. Therefore, evaluation of the optimal economic outcome must start with optimization of the well for efficiency and half-length followed by spacing. Completion efficiency can be summarized as increasing net present value per barrel while maintaining efficient use of the land available for development.
Hydraulic-Fracture Modeling
Two modeling approaches were taken to estimate proppant placement. One of the established 3D planar-fracture models was used in conjunction with a finite-element geomechanical model. Both of these models present challenges in their interpretation. 3D planar models seek to model the effects within the fracture accurately. While stress shadowing between clusters is considered, the compromise is that the model requires the fracture to be always planar, an assumption almost always never fully met. Complexity seen often makes half-length achieved by a planar-fracture model optimistic; therefore, this was used as a best case for length of propped section in the simulation work. In addition, half-lengths predicted from the model were compared with half-lengths calculated from rate-transient analysis and tracer surveys taken when wells were stimulated. The finite-element geomechanical model was used to look at stress changes from offset wells and to optimize cluster spacing. The downside to the finite-element geomechanical models commercially available is their inability to model the proppant placement within the created fracture and other critical parameters such as width and height growth. Therefore, these two approaches complement one another, and are in fact both rather limited without other information.
Stochastic Simulation
Multiple simplistic simulation models were history matched to existing production wells. For each well, key reservoir uncertainties, including effective permeability, permeability reduction with pressure, original hydrocarbons in place, and formation compressibility, were allowed to vary. Porosity was modeled as effective porosity, excluding the volume of adsorbed hydrocarbons estimated to be present.
The models were defined with an effective permeability that accounts for the possible influence of natural fractures. Permeability reduction with pressure was found to be a critical parameter in the estimation of ultimate recovery. Core-based measurements of permeability vs. net confining stress were converted to permeability vs. pressure and were included in the simulations in the form of a gamma (or permeability-modulus) function.
Wells selected for history matching ranged from dry gas to near-critical gas/condensate. Fluid properties were modeled with equation-of-state models, tuned to available pressure/volume/temperature data and extrapolated from sampled wells on the basis of trend maps of produced-condensate yield.
Completion parameters, including fracture conductivity and half-length of dominant and minor fractures, were also tested. For each run, the fracture geometry as predicted from the hydraulic-fracture model was preserved, while the length, conductivity, and fracture efficiency were varied.
Initial fracture conductivity was estimated from fracture width and proppant concentration predicted by hydraulic-fracture modeling. Initial conductivity was then modified to account for changing stress and production conditions, including embedment, crushing, and spalling effects. The models were found to be relatively insensitive to the predicted range of fracture conductivity because of the large contrast in flow capacity between the hydraulic fractures and the formation in all scenarios.
Assisted-history-matching methods were used to explore the solution space within the defined uncertainty ranges and identify all possible parameter sets that can match production history (Fig. 3). The alternative history-matched cases were used as the basis for predictive modeling, enabling a robust range of forecast outcomes.
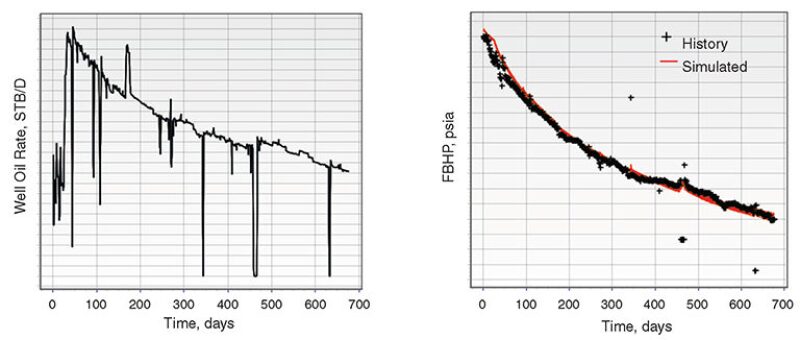
The history-matching process provided a means of calibrating both the geocellular and hydraulic-fracture models. The original uncertainty estimates were narrowed where the full range of inputs was demonstrated not to honor the field data. Alternative reservoir descriptions, validated through history matching, provide the basis for well optimization. For each well, a selection of history-matched realizations was used to test alternative completion designs and the impact of well spacing.
This article, written by JPT Technology Editor Chris Carpenter, contains highlights of paper SPE 168973, “Unconventional-Asset-Development Work Flow in the Eagle Ford Shale,” by David Cook, Kirsty Downing, Sebastian Bayer, Hunter Watkins, Vanon Sun Chee Fore, Marcus Stansberry, Saurabh Saksena, and Doug Peck, BHP Billiton Petroleum, prepared for the 2014 SPE Unconventional Resources Conference—USA, The Woodlands, Texas, USA, 1–3 April. The paper has not been peer reviewed.