This paper presents the implementation of an approach for improving oil recovery by water-injection optimization using injection-control devices (ICDs) in unconventional reservoirs. In late 2016, a trial campaign began in southeastern Saskatchewan that applied ICDs in relatively low-flow-rate environments to offset production decline and improve recovery. Early-term results show that an improvement in oil recovery greater than 25% over typical waterflood configurations is possible.
Introduction
To offset production decline caused by normal pressure decline and reservoir drainage, secondary recovery using water injection has been administered in three Saskatchewan tight oil plays. This study will be focused on the Bakken formation, the area with the most-complete historical data set available to the authors.
The original completion and well-spacing plan has resulted in occasional direct water channeling from injection to production wells.
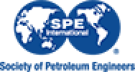