Multiwell modeling of shale plays is not performed frequently, mainly for two reasons: The requirements to capture the behavior of multiple horizontal wells with multiple hydraulic fractures could be computationally prohibitive, and the wells in extremely-low-permeability formations would have minimal interaction. Production data collected from shale plays, however, have shown that horizontal wells may interfere with each other during production. Therefore, in projects in which a main objective is well spacing or completion optimization, a comprehensive multiwell reservoir-simulation study is required.
Introduction
Single-well reservoir modeling has been used extensively to perform reservoir simulation in shale formations. In the appraisal phase of liquids-rich shale (LRS) reservoir systems, more detail may be required to capture petrophysical and stratigraphic features that can potentially affect production performance. During this phase, single-well conceptual models with a certain degree of detail can be sufficient for modeling purposes and economic analysis. However, if the presence of geological features (e.g., faults, fracture corridors, contrast of areal and vertical distribution of natural-fracture intensity) is believed to affect the economic evaluation of a certain area of interest, then a more-detailed geological model is required and multiple wells may have to be incorporated in the models.
Performing multiwell, or sector, modeling of shale formations can present several challenges:
- An adequate description of permeability and water saturation may be difficult to attain because the original values are considerably altered in the stimulated-rock-volume (SRV) region after the fracturing treatments.
- The capture of the transient flow observed in LRS formations and the large pressure gradients around the fracture planes requires extensive grid refinement.
- The large drawdown necessary to produce from these ultralow-permeability formations can make pressure/volume/temperature (PVT) sampling a poor representation of the original reservoir conditions.
- The interaction of hydraulic fractures with natural fractures or the development of fracture networks can be very difficult to model.
Seismic
The application of seismic for reservoir simulation of LRS plays goes beyond the classical reservoir-simulation workflow; seismic processing, inversion, and interpretation can provide important reservoir-property distributions that are key parameters for reservoir simulation and production analysis.
A variety of seismic attributes can be used to predict the intensity and orientation of probable pre-existing natural fractures and the correlation with microseismic responses. This can be used to build discrete-fracture-network (DFN) models and patterns of hydraulic-fracture propagation and interaction with natural fractures and to constrain the SRV size and shape.
Acoustic impedance can be used to generate porosity and pore-pressure distributions within a target shale region. The logic is that acoustic impedance is a linear function of density, and density can be correlated with porosity from a petrophysical model.
Seismic interpretation calibrated with rock-property models is also used to provide rock-property distribution in a specific area to constrain and validate production from reservoir-simulation models.
PVT Sampling and Analysis
The extremely low permeability of shale formations requires large drawdowns to produce hydrocarbons commercially, and, typically, the early production at the lowest possible drawdown is nearly 100% water, recovered from the fracturing treatment. The large drawdowns applied also produce significant liquid losses, especially in the volatiles/condensate window; therefore, the low liquid recovery is strongly dependent on PVT behavior.
In that situation, one possible solution is to combine advanced PVT modeling with single-well reservoir simulation to match production performance using fluid initialization parameters as key history-matching variables.
Cartesian Grids, Tartan Grids, and Unstructured Gridding
The production behavior from shale formations can be in transient flow for months or years because of the extremely low matrix permeability. When hydraulic fractures are placed in the formation, the flow regimes can vary from linear flow to bilinear flow in the first months or years until the pressure transient reaches determined boundaries (fracture-stage boundaries, boundaries at the tips of the fractures, or reservoir boundaries) after several years of production. The hydraulic fractures introduce large pressure gradients at the fracture faces and tips because of the large contrast between the matrix and fracture permeabilities. Therefore, special grid refinement is required around those regions in order to capture the characteristic flow regimes.
Cartesian Grids With Local Grid Refinement (LGR). LGR can be applied around the main hydraulic-fracturing planes in order to capture transient flow. This approach adds more cells, increasing the running time; however, it is relatively simple to implement and can make the incorporation of SRVs from microseismic interpretation easier.
Tartan Gridding. In a tartan grid, the cells are globally distributed in the reservoir following a logarithmic or geometric propagation around the fracture planes, fracture tips, and well lateral length. This gridding type is a preferred method because it allows for the capture of transient flow properly and for optimization of the number of cells, reducing running time. However, the process of building tartan grids for multiwell modeling can be very laborious because of a lack of this capability in most of the geomodeling software packages currently available.
Unstructured Gridding. Unstructured gridding is the ideal gridding method because it solves all the issues related with nonparallel laterals and nonorthogonal fracture planes; and it fits very well with complex fracture simulators because it captures complex fracture geometries. However, this type of gridding requires important reformulation of flow modeling, and most commercial reservoir simulators do not have this capability fully implemented yet.
Hydraulic-Fracturing Modeling
Hydraulic-fracturing modeling is not commonly included within conventional reservoir-simulation workflows. However, for reservoir-simulation studies of shale formations, a fair understanding of the propagation of hydraulic fractures and their interaction with pre-existing natural fractures should be considered an integral part of the reservoir-simulation workflow in order to estimate or constrain the size and shape of the SRV.
Some simulators with the ability to predict the propagation of complex fracture networks have been released commercially. These simulators have capabilities to build DFN models or can import DFN models from geomodeling software packages. The first approach uses classical linear elastic approaches to model the propagation of the hydraulic fracture from its initiation and vertical propagation into surrounding layers or into natural fractures. The interaction with the natural fractures is modeled on the basis of propagation of fracturing-fluid pressure and leakoff into the connected -natural-fracture system. This propagation model is constrained by the state of in-situ stresses, the orientation and geometry of the pre-existing natural-fracture system, rock properties, and the interpretation of microseismic monitoring (Fig. 1). The second approach models the fracture propagation, fluid flow, and proppant transport in a complex fracture network. The interaction between the created hydraulic fracture and the pre-existing natural-fracture system is modeled with an analytical model that also considers the mechanical interaction with adjacent fractures as a result of multistage fracturing (Fig. 2). Although this method currently is limited to single-well modeling, it can provide a relatively good understanding of the size and shape of the SRV.
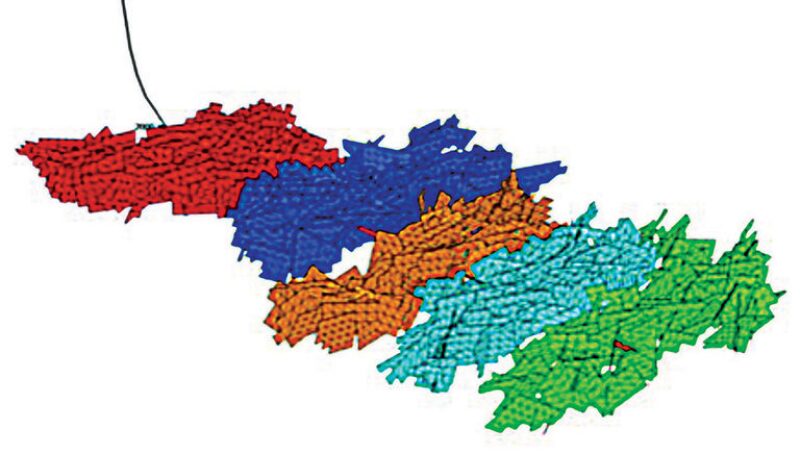
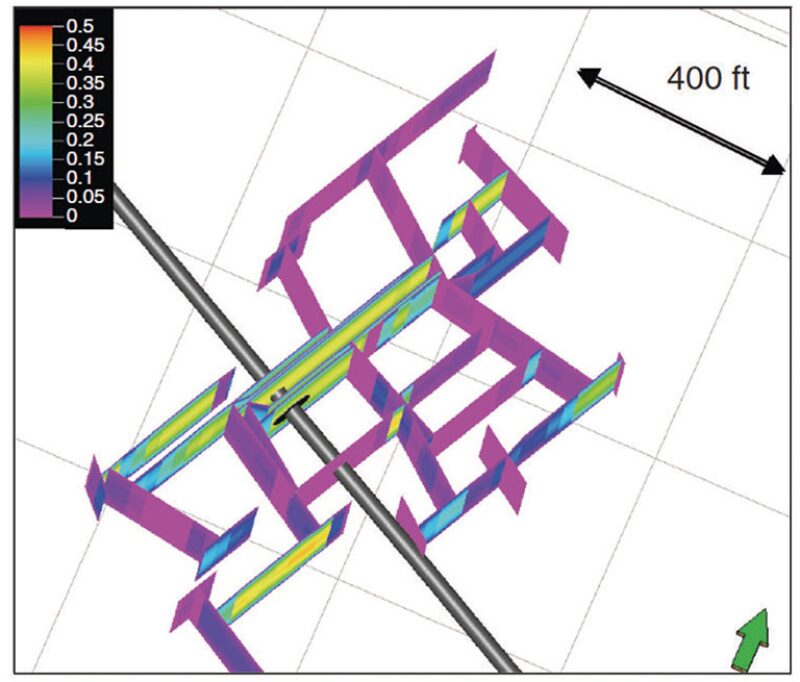
Microseismic Monitoring
Microseismic events are seismic energy released from shear failures away from the hydraulic fracture and along reactivated/induced natural fractures, if they are present. Therefore, microseismic monitoring frequently displays a broad cloud of microseismic events that is not necessarily associated with connected complex fracture networks.
A complex fracture network follows the physical interaction of the hydraulic fractures with the pre-existing natural-fracture system, and its development is conditioned by the state of the in-situ stresses (maximum and minimum horizontal stresses), the orientation and geometry of the natural fractures, rock properties, fluid properties (e.g., pressure and rheology), and the natural-fracture interface properties (e.g., friction coefficient, cohesion, and permeability).
When properly interpreted and combined with geomodels and production performance, microseismic monitoring can be a very useful tool for multiwell spacing and hydraulic-fracturing optimization.
Proposed Reservoir-Simulation Workflow
Fig. 3 presents a workflow to help in the process of multiwell reservoir simulation for LRS formations. This workflow presents some departures from the classical reservoir-simulation workflow for conventional reservoirs. Shale characterization, complex-fracture modeling, and microseismic interpretation have been incorporated to address the effect of several static and dynamic factors that are critical for reservoir simulation of LRS formations.
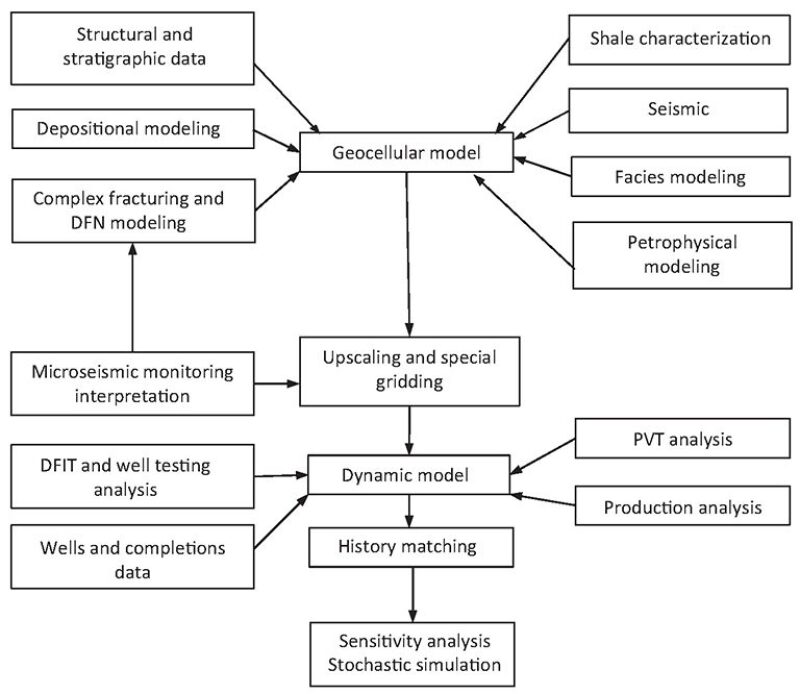
Conclusions
- Multiwell (sector) reservoir simulation can be performed with some acceptable degree of confidence with adequate modeling (or a fair understanding) of the propagation of hydraulic fractures in the shale formation.
- Although conceptual models can be used in many cases, a more-detailed geological description may be required for well-spacing and fracture-stage-spacing studies when geological features are believed to affect the production performance.
- PVT analysis and modeling are critical for the simulation of LRS formations.
- The reservoir-simulation workflow for LRS formations should incorporate shale characterization, complex-fracturing and DFN modeling, and microseismic-monitoring interpretation.
- Shale characterization plays an important role in the reservoir-simulation process of LRS formations because it provides important insights to constrain and guide the building process of the geocellular and reservoir-simulation models.
- The characteristic transient flow of these formations requires special gridding features. This can be a very complicated task for multiwell modeling in some of the commercial geomodeling software packages. However, this can be accomplished by use of external applications for tartan gridding or by use of more-sophisticated unstructured-gridding capabilities when available.
- Although this can be considered a simple basic observation for conventional reservoirs, the formation thickness or the ratio of fracture height to formation thickness is a critical factor for reservoir simulation of LRS formations.
This article, written by Special Publications Editor Adam Wilson, contains highlights of paper SPE 169025, “A Study of Dynamic and Static Factors That Are Critical to Multiwell Reservoir Simulation of Liquids-Rich Shale Plays,” by Carlos Miranda, SPE, and Huade Yang, Marathon, prepared for the 2014 SPE Unconventional Resources Conference—USA, The Woodlands, Texas, USA, 1–3 April. The paper has not been peer reviewed.